Does a ketogenic diet confer the benefits of butyrate without the fibre?
Tenuous arguments from fibre apologists
According to many plant-eating enthusiasts, we must eat fibre to be healthy for the following reasons:
Note:
These are not the only arguments people make for eating fibre. These are only reasons related to butyrate.
- Fibre is the only way to get butyrate.
- Butyrate prevents colon cancer.
- Butyrate in the colon treats colitis.
- Butyrate is the preferred fuel of the colonocyte, therefore it is essential.
- Without butyrate your colon cells will die off.
Of the above statements, only one of them seems well-justified to me, but it also seems irrelevant. Let’s start from the end.
Myth: Without butyrate your colon cells will die off.
This idea (a quote from Wikipedia) seems to to be an exaggerated interpretation of a study by Donohoe et al.. The authors are studying germ-free mice, who don’t, of course, have bacteria synthesising butyrate. They describe what looks to them like impaired colon cell energetics in the mice and ultimately autophagy upregulation, meaning the cells are eating themselves. They reverse these effects with butyrate. I’ve already written about some of the curious paradoxes inherent in the study. To summarise, other studies consistently find germ-free mice to be healthier than wild mice by many a measure, including appearing to be more energetic, and living longer. There seems to have been a conflation of cell energy with mitochondrial energy, by not looking for mitochondrial density changes. So, I’m not convinced the butyrate made things better.
Likewise, the reported evidence of autophagy (increased autophagosomes attributed to upregulation of AMPK), insofar as it indicates autophagy, could equally be a desirable result, given the role of autophagy in maintaining healthy tissues. See, e.g. [Miz2011]. Certainly unrestrained autophagy, with no homeostatic mechanism, should result in total loss of tissue, but that doesn’t seem to happen with the germ-free mice. Germ-free rodents have freakishly large caecums, and somewhat reduced small intestines, but so far as I can tell, no colon abnormalities worth mentioning. For an extensive review of the data already available in 1971 on germ-free animals, including the structure and function of various organs, see The gnotobiotic animal as a tool in the study of host microbial relationships.
In any case, if the colons of germ-free mice are at any disadvantage there are clearly more differences that might be attributable to than mere lack of butyrate. Are there other reasons to worry about colon cells that don’t get any?
Myth: Butyrate is the preferred fuel of the colonocyte, therefore it is essential.
If you haven’t read my thoughts on the term “preferred”, the point is that what a cell will consume first isn’t necessarily the fuel that is the healthiest, though it certainly can be. Other reasons could be to get rid of it, or to access the metabolites. I’m not really suggesting that butyrate is toxic to colon cells. (Though as soon as that thought occurred to me I looked for evidence that it can be, which, of course there is [Pen2007]. Apparently it can accumulate due to maldigestion or bacterial overgrowth and cause serious epithelial damage. But I digress.) All I’m saying is that habitual heavy use doesn’t imply something is needed. The same argument has been made about glucose in the brain, and we all know that the brain actually needs only a very small amount of glucose, if β-hydroxybutyrate is in good supply. It’s still possible that other fuels are as good or better than butyrate for the colonocyte.
Myth: Butyrate in the colon treats colitis.
Normally, colonocytes do metabolise butyrate, mostly into CO2 and ketone bodies, but this is impaired in ulcerative colitis [Roe1980], [Roe1993], [Ahm2000], such that ketogenesis is is inversely proportional to the severity of the disease [Roe1980].
This impairment may explain the mixed results in treatments involving butyrate. Some researchers have tried to treat colitis by adding more butyrate for substrate, by enema. Perhaps unsurprisingly, that has not met with much success. Or has it? I read a somewhat confusing review [Mal2015] that has several citations in it that don’t appear to line up with the claims preceded by the citations, including citing the same paper that I’ve cited above (Roe1980), as showing “that restoration of butyrate levels by intracolonic infusion treats UC”, which I can find no mention of in the paper, and citing a single paper twice, ([Ham2010]), once to say that enemas had very limited effect (which I think is correct) and once, later, to say it was a “well demonstrated” “cure”. These are probably just simple citation errors on my part or theirs.
There have been some successes using enemas, but the results are mixed [Ham2008]. Insofar as there are successes, it is worth noting that the butyrate was taken in by rectal cells, not colon cells, and so the effect was post-absorptive. In other words, it must have come systemically. In fact, when the butyrate is applied directly to impaired cells it seems to worsen the situation. These points are noted in the review, and motivates their own contribution.
The researchers used intraperitoneal injections of butyrate to apparently almost completely restore colonocyte integrity in rodent models of colitis. At face value, this would suggest that it is not the butyrate that helped, but a metabolite of butyrate, i.e. ketone bodies, since peritoneal injections normally pass through the liver [Tur2011]. If it’s systemic ketone bodies we want, we know how to do that! Also, this method is rarely used in humans, so it may not be easy to make any practical use of. In any case, none of this would suggest that eating plant fibre will help colitis in any way, given that the issue appears to depend on inability to use the butyrate.
Ulcerative Colitis UC and Crohn’s Disease (CD) constitute the Inflammatory Bowel Diseases (IBD). There is not clear evidence that fibre intake helps with IBD, and in fact, “low residue” or “low fibre” diets are usually recommended (see below). In case you were wondering, “residue” means anything that survives digestion, and comes all the way through the intestines. That includes fibre , but also microorganisms, and secretions and cells shed from the alimentary tract.
While there are studies that support the benefit of fibre in IBD, there are others showing harm. The evidence is mixed enough to be called weak and inconclusive [Kap2016].
Anecdotes such as the “Crohn’s Carnivore” suggest a different solution might hold for some:
“Eight years ago I decided to eat nothing but meat for a year. Now I have a perfectly normal colon. If those two events are indeed correlated, and someone could figure out exactly how, a whole lot of people would be able to find relief from a terrible disease.”
That experience runs both with and possibly against current dietary guidelines for IBD. In a 2011 review [Bro2011], the authors show that most guidelines advise low fibre intake, especially during flares. Some also advise low fat intake, and in particular, to eat lean meat. I’m not sure whether the Crohn’s Carnivore was eating lean or fatty meat during his year of healing. At first blush, the low fat advisory looks like just another “extra-mile” kind of recommendation, in which guideline writers are throwing in other ideas about healthy diet for good measure. However, they state that it comes from the reported reactions of some patients. They also cite patient surveys which list meat as a provoking food in 25% of respondents. (The most common response was vegetables, at 40%). One wonders if there are conflations. Later, the authors specifically say that there is little to support or refute a low fat recommendation.
Another anecdote, this time elevated to “case study” level, because physicians penned it, comes from the Evolutionary Medicine Working Group, in Budapest, Hungary [Tot2016]. They report complete resolution of symptoms in a child with Crohn’s and cessation of medications from an essentially meat-only diet. The exception was that patient was allowed some honey, but it was low enough that ketosis was maintained. This was a 2:1 fat:protein diet, so definitely not low fat. The child had previously tried low fat, low fibre, and several medications without improvement. It is interesting to note that even one dose of “paleo approved” fibre caused a flare up.
“Given the patient’s severe condition upon the first visit the paleolithic ketogenic diet was started in the strictest form thus containing no vegetables and fruits at all. Such a diet may first sound restrictive but our previous experience indicate that a full fat-meat diet is needed in the most severe cases of Crohn’s disease. In addition, our experience shows that even a single occasion of deviation from diet rules may result in lasting relapse. This was the case in the present patient too where breaking the strict rules (eating the “paleo cakes”) resulted in a thickening of the bowel wall. Based on our experience this is due to the components of the popular paleolithic diet including coconut oil, oil seeds and sugar alcohols which may trigger inflammation.”
In other words, a fibre-free ketogenic diet appears help IBD more than a diet including fibre, even a ketogenic diet including fibre.
Maybe: Butyrate prevents colon cancer.
The idea that butyrate might be protective of colon cancer seems to have started in the 1980s (see, e.g., [Sen2006].
This area of research is extensive, and I am by no means an expert. If you haven’t guessed, that butyrate has a protective effect on colon cancer is the one statement I think is entirely defensible.
It’s not known exactly how butyrate exerts its protective effects, but some mechanisms held to be important are also induced by β-hydroxybutyrate. For example butyrate’s histone deacelytase (HDAC) inhibition is considered an important mechanism [Hin2002], [Blo2011]. β-Hydroxybutyrate is also an HDAC inhibitor [Shi2013].
Gpr109a receptor activation is a recently identified mechanism [Sin2014]. Gpr109a has many aliases, including hydroxycarboxylic acid receptor 2 (HCA2) or niacin receptor 1 (NIACR1), and HM74a/PUMA-G. Gpr109a is activated by β-hydroxybutyrate [Tag2005], [Rah2014], [Gam2012]. It is sometimes simply called the β-hydroxybutyrate receptor.
In fact, the argument behind the relevance of the Gpr109a discovery is just as strong an argument for a ketogenic diet as for eating fibre! (This sentence is incorrect. See Edit.) That is, the researchers demonstrated that butyrate could substitute for niacin in activating these receptors, and that just as niacin activation of Gpr109a in fat cells is protective of cardiovascular disease, it may also be in diseases of the colon, and this argues for eating fibre to substitute for pharmalogic doses of niacin. From a press release:
“We think mega-doses of niacin may be useful in the treatment and/or prevention of ulcerative colitis, Crohn’s disease, and colorectal cancer as well as familial adenomatous polyposis, or FAP, a genetic condition that causes polyps to develop throughout the gastrointestinal tract”
…
“Research teams at GlaxoSmithKline and the University of Heidelberg, Germany showed in 2003 that Gpr109a receptors on the surface of fat cells mediate the protective cardiovascular effect of niacin, including increasing good cholesterol, or HDL, while decreasing levels of disease-producing LDL. Their search for other activators identified butyrate, which led Ganapathy to find that not only is the Gpr109a receptor expressed on the surface of colon cells, but that with sufficient fiber intake, butyrate levels in the colon can activate it.”
[Edit] 2018-01-04: A critic pointed out that the cell receptors for SCFAs are facing the lumen, and therefore argued that beta-hydroxybutyrate from the portal side would be irrelevant. Indeed, the researchers using niacin also assume that the extremely high dose of niacin does not act sytemically, but rather reaches the lumen because of the super-high doses. So the statement I made above, about the argument for beta-hydroxybutyrate being equal to that for niacin is not correct. The argument still stands that the beta-hydroxybutyrate metabolites activating targets inside could be where the majority of the benefits of butyate come from. That is where the HDAC inhibition occurs and where the immune cell receptors are. At least one research group agrees with my speculation that the interior metabolites may be important for the effect [Siv2017]
” “As the cell-surface receptors for SCFAs are located on the lumen-facing apical membrane of colonic epithelial cells (see below), the luminal concentrations of these agonists are physiologically relevant. SCFAs are low-affinity agonists for these receptors, and the normal luminal concentrations of these bacterial metabolites are in the millimolar levels, sufficient to activate these receptors from the luminal side. However, some of the molecular targets for these metabolites are either inside the cells (e.g., HDACs) or on the surface of the immune cells located in the lamina propria. Therefore, concentrations of these metabolites inside the colonic epithelial cells and in the lamina propria are relevant to impact these molecular targets. The intracellular target HDAC is inhibited by butyrate and propionate at low micromolar concentrations. There are effective transport systems for SCFAs in the apical membrane of colonic epithelial cells (e.g., proton-coupled and sodium-coupled monocarboxylate transporters) [47], thus making it very likely for these SCFAs to reach intracellular levels sufficient to inhibit HDACs. Even though the luminal concentrations of SCFAs are in the millimolar range, it is unlikely that they reach lamina propria at significant levels to activate the cell-surface receptors present on the mucosal immune cells. These metabolites are present only at micromolar levels in the portal blood [57], indicating that they undergo robust metabolism inside the colonic epithelial cells. This raises the question as to the physiological relevance of these bacterial metabolites to the activation of the cell surface SCFA receptors in immune cells located in the lamina propria. With regard to this issue, it is important to note that colonic epithelial cells are highly ketogenic; they use acetate and butyrate to generate the ketone body β-hydroxybutyrate [58]. This ketone body is released from the cells into portal blood. As β-hydroxybutyrate is 3–4 times more potent than butyrate in activating its receptor GPR109A, it can be speculated that the colon-derived ketone body is most likely involved in the activation of the SCFA receptor in mucosal immune cells.”
Moreover, see the preliminary systemic evidence below.
Interestingly, as in the case of colitis, colorectal cancer appears to involve a dysfunction in ability to use butyrate. Specifically, there are detrimental changes in membrane transport that reduce its entry into the cell [Gon2016]. Therefore, it’s unclear that once the disease process has begun, increased fibre intake will be of any use. Beta-hydroxybutyrate in the bloodstream, however, might.
There is at least some preliminary evidence that butyrate in the bloodstream has similar effects on intestinal tissue as butyrate coming from the colon itself [Kor1990], [Rol1997], [Bar2004], as does infusion of glutamine and acetoacetate, another ketone body [Rom1990]. Ketogenic diets do increase blood acetoacetate. If bloodstream infusion of butyrate is as effective as absorption of butyrate in the intestines in protecting colon cells from degradation, then it seems reasonable to hypothesise that β-hydroxybutyrate in the bloodstream would also have this effect.
These common mechanisms suggest that much or even all of the benefits obtainable by butyrate are equally achievable simply through ketogenic diets, making additional butyrate in the context of a ketogenic diet potentially superfluous.
Myth: Fibre is the only way to get butyrate.
Even though it seems likely that a fibre-free ketogenic diet is not only sufficient for colon health, but better for treating colon disease, we might feel cautious about going without the butyrate from fibre, given the dire pronouncements from nutritional scientists. Is there any other way to get butyrate? The most significant food source, butter, doesn’t give much. Only about 3-4% of butter is butyric acid. According to [Sen2006] we produce >200mmol per day. That would take about a pound of butter!
Stepping back, it should be obvious that carnivores such as felines and canines provide an important source of data relevant to this question. Carnivores have colons, and they are not normally in ketosis unless food is scarce. Either their colons don’t need butyrate, or they are getting sufficient butyrate from some other source. As it happens, there are microbes that ferment amino acids in to short chain fatty acids (SCFAs), including butyrate. Carnivores are known to get “animal fibre” from their prey. That is, amino acids from incompletely digested animal parts reach their colons and are fermented. In particular, in cheetahs, casein, collagen, and glucosamine have been shown to result in butyrate production comparable to fructo-oligosaccharides [Dep2012].
Beyond poorly digested animal sourced fibre, many amino acids are fermented into SCFAs, including butyrate [Ras1988], and these amino acids are abundant in human intestines and colons and are fermented there [Vit2014], [Dai2015], [Nei2015], [Wie2017]. I was unable to determine how much butyrate this would account for.
I did find research comparing the SCFA levels produced in dogs under conditions of high fibre vs. meat alone showing that they produced almost as much VFA (another word for SCFA) in their colons eating meat alone [Ban1979].
In any case, we certainly do generate butyrate in the absence of dietary fibre.
In sum
Although many in the medical community consider butyrate an essential fuel for colon cells, there may be a parallel to glucose and brain cells, in that some or all of this functionality could be replaceable with β-hydroxybutyrate. This idea is supported by these observations:
- Carnivores and even germ-free mice have intact, working colons without contributions from fibre-derived butyrate, so it stands to reason that humans may not need it either.
- Although not discussed in this post, some recent societies thrived on animal-based diets with little and infrequent plant intake.
- β-hydroxybutyrate triggers many of the same mechanisms that butyrate does; those very mechanisms thought to explain its role in preventing colon cancer and the intestinal degradation seen in diseased colons or the colons of those receiving reduced fibre diets to promote bowel rest.
- β-hydroxybutyrate may even be the pathway through which butyrate exerts its beneficial effects, given that it is a direct metabolite of butyrate, and that systemic butyrate appears to be as effective or even more effective in treating colitis, than direct application of butyrate to the cells.
- Even without eating fibre, our intestinal microbes produce butyrate from amino acids. If systemic ketone bodies supplant or even just reduce the need for butyrate, amino acid derived butyrate may supply this need, even if the quantities turn out to be less than we would get from fibre.
End-to-end citations
[Ahm2000] Evidence type: non-human animal experiment
Butyrate and glucose metabolism by colonocytes in experimental colitis in mice.
Ahmad MS, Krishnan S, Ramakrishna BS, Mathan M, Pulimood AB, Murthy SN.
Gut. 2000 Apr;46(4):493-9.
“Abstract
“BACKGROUND/AIMS:
“Impaired colonocyte metabolism of butyrate has been implicated in the aetiopathogenesis of ulcerative colitis. Colonocyte butyrate metabolism was investigated in experimental colitis in mice.
“METHODS:
“Colitis was induced in Swiss outbred white mice by oral administration of 4% dextran sulphate sodium (DSS). Colonocytes isolated from colitic and normal control mice were incubated with [(14)C]butyrate or glucose, and production of (14)CO(2), as well as of intermediate metabolites (acetoacetate, beta-hydroxybutyrate and lactate), was measured. The effect of different substrate concentrations on oxidation was also examined.
“RESULTS:
“Butyrate oxidation (micromol/h per mg protein; mean (SEM)) was significantly reduced in DSS colitis, values on day 7 of DSS administration being 0.177 (0.007) compared with 0.406 (0.035) for control animals (p<0.001). Glucose oxidation (micromol/h per mg protein; mean (SEM)) on day 7 of DSS administration was significantly higher than in controls (0.06 (0.006) v 0.027 (0.004), p<0.001). Production of beta hydroxybutyrate was decreased and production of lactate increased in DSS colitis compared with controls. Increasing butyrate concentration from 10 to 80 mM enhanced oxidation in DSS colitis (0.036 (0.002) to 0.285 (0.040), p<0.001), although it continued to remain lower than in controls. Surface and crypt epithelial cells showed similar ratios of butyrate to glucose oxidation. When 1 mM DSS was added to normal colonocytes in vitro, it did not alter butyrate oxidation. The initial histological lesion of DSS administration was very patchy and involved crypt cells. Abnormal butyrate oxidation became apparent only after six days of DSS administration, at which time histological abnormalities were more widespread.
“CONCLUSIONS:
“Colonocyte metabolism of butyrate, but not of glucose, is impaired in DSS colitis, and may be important in pathophysiology. Histological abnormalities preceded measurable defects in butyrate oxidation.”
[Ban1979] Evidence type: non-human animal experiment
Sites of organic acid production and patterns of digesta movement in the gastrointestinal tract of dogs
Banta, C. A., Clemens, E. T., Krinsky, M. M., and SheiIy, B. E., 1979,
J. Nutr. 109:1592-1600.
“Two commercial type diest, one a cereal based dry food, the other a fortified all meat canned food were fed to male and female adult beagle dogs to evaluate effects of diet on rate of digesta passage and organic acid concentration along the gastrointestinal tract. […]
Concentrations of VFA were highest in the cecum and colon and were not significantly affected by diet.”
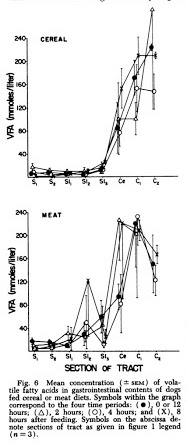
“Symbols on the abscissa denote sections of tract as follows: cranial stomach ( Si ) ; caudal stomach ( 82) ; proximal ( Sii ), middle (SI2) and distal (SL) thirds of the small intestine; cecum (Ce); and proximal (Ci) and distal (C«) halves of the colon ( n = 3 ).”
[…]
“It was surprising to see high concentrations of VFA produced in the lower gut of dogs fed the meat diet. It was logical to assume that liver and muscle glycogen could serve as the fermentable substrate for lactate production in the stomach, but most of this should have been digested and absorbed by the small intes tine. Another possible source of ferment able substance which could survive passage through the small intestine is the protein-polysaccharides of the connective tissue ground substance found in abundance in the meat by-products and whole ground chicken. The ground substance is made up of chondroitin sulfates and hyaluronic acid. The polysaccharide portion of these substances is composed of long chains of disaccharide units consisting of glucosa- mine or galactosamine and glucuronic acid. The linkages of these polysaccharides are not such that they can be cleaved by the endogenous digestive enzymes found in the gut but they could be split by microbial enzymes.”
[Bar2004] Evidence type: non-human animal experiment
Supplementation of total parenteral nutrition with butyrate acutely increases structural aspects of intestinal adaptation after an 80% jejunoileal resection in neonatal piglets.
Bartholome AL1, Albin DM, Baker DH, Holst JJ, Tappenden KA.
JPEN J Parenter Enteral Nutr. 2004 Jul-Aug;28(4):210-22; discussion 222-3.
“BACKGROUND:
“Supplementation of total parenteral nutrition (TPN) with a mixture of short-chain fatty acids (SCFA) enhances intestinal adaptation in the adult rodent model. However, the ability and timing of SCFA to augment adaptation in the neonatal intestine is unknown. Furthermore, the specific SCFA inducing the intestinotrophic effects and underlying regulatory mechanism(s) are unclear. Therefore, we examined the effect of SCFA supplemented TPN on structural aspects of intestinal adaptation and hypothesized that butyrate is the SCFA responsible for these effects.
“METHODS:
“Piglets (n = 120) were randomized to (1) control TPN or TPN supplemented with (2) 60 mmol/L SCFA (36 mmol/L acetate, 15 mmol/L propionate and 9 mmol/L butyrate), (3) 9 mmol/L butyrate, or (4) 60 mmol/L butyrate. Within each group, piglets were further randomized to examine acute (4, 12, or 24 hours) and chronic (3 or 7 days) adaptations. Indices of intestinal adaptation, including crypt-villus architecture, proliferation and apoptosis, and concentration of the intestinotrophic peptide, glucagon-like pepide-2 (GLP-2), were measured.
“RESULTS:
“Villus height was increased (p < .029) within 4 hours by supplemented TPN treatments. Supplemented TPN treatments increased (p < .037) proliferating cell nuclear antigen expression along the entire intestine. Indicative of an antiapoptotic profile, jejunal Bax:Bcl-w abundance was decreased (p = .033) by both butyrate-supplemented TPN treatments, and ileal abundance was decreased (p = .0002) by all supplemented TPN treatments, regardless of time. Supplemented TPN treatments increased (p = .016) plasma GLP-2 concentration at all time points.
“CONCLUSIONS:
“Butyrate is the SCFA responsible for augmenting structural aspects of intestinal adaptations by increasing proliferation and decreasing apoptosis within 4 hours postresection. The intestinotrophic mechanism(s) underlying butyrate’s effects may involve GLP-2. Ultimately, butyrate administration may enable an infant with short-bowel syndrome to successfully transition to enteral feedings by maximizing their absorptive area.”
[Bro2011] Evidence type: review
Existing dietary guidelines for Crohn’s disease and ulcerative colitis.
Brown AC, Rampertab SD, Mullin GE.
Expert Rev Gastroenterol Hepatol. 2011 Jun;5(3):411-25. doi: 10.1586/egh.11.29.
“In terms of existing guidelines for dietary modifications, three suggested limiting dairy if lactose intolerant, two suggested limiting excess fat, one indicated decreasing excess carbohydrates, and five suggested avoiding high-fiber foods, especially during flares. The question of whether or not to use probiotics continues to be debated.”
[…]
“Reducing high-fiber foods during symptoms appears to have generated the most support in the dietary guidelines. It may be important to communicate to IBD patients that high-fiber foods are not recommended, especially for those with CD, during flares or in the presence of active disease states, fistulas or strictures. There appears to be a tendency among the dietary guidelines to restrict foods such as raw fruits, raw vegetables, beans, bran, popcorn, seeds, nuts, corn hulls, whole grains, brown rice and wild rice. Although not mentioned, raw salads would also fall into this category.”
[…]
“Some patients with IBD react to excess dietary fat and perhaps this is where the recommendation is derived. Few research studies are available to support or refute such a recommendation. The topic needs further investigation because patients with malabsorption may be at risk of not obtaining their necessary essential fatty acids. Perhaps saturated fats should be limited, with more of an emphasis on more healthy fat intakes.”
[Dai2015] Evidence type: review
Amino acid metabolism in intestinal bacteria and its potential implications for mammalian reproduction
Zhaolai Dai Zhenlong Wu Suqin Hang Weiyun Zhu Guoyao Wu
MHR: Basic science of reproductive medicine, Volume 21, Issue 5, 1 May 2015, Pages 389–409
“Recent studies with the human colonic bacteria have shown that protein- and AA-fermenting bacteria are abundant and diverse in the colon. The abundance of the AA-fermenting bacteria in the large intestine is very high and their number can reach up to 1011 per gram dry feces (Smith and Macfarlane, 1998). Using the traditional plate counting technique, the authors have also reported that the dominant bacterial species for the utilization of single AA or pairs of AA are very different. For instance, Clostridium bifermentans is the predominant bacteria for the utilization of lysine or proline, and pairs of AA (e.g. phenylalanine/leucine, isoleucine/tryptophan and alanine/glycine), whereas Peptostreptococcus spp. bacteria are predominant for the utilization of glutamate or tryptophan. Many species of bacteria utilize the same AA as substrates for growth (Smith and Macfarlane, 1998). Overall, bacteria belonging to the Clostridium spp. dominate in AA fermentation in the human large intestine, but other bacterial species, such as Fusobacterium spp., Bacteroides spp., Veillonella spp., Megasphaera elsdenii and Selenomonas ruminantium, may also be important for AA metabolism in the large intestine (Smith and Macfarlane, 1998; Dai et al., 2011).”
[Dep2012] Evidence type: non-human animal experiment
Fermentation of animal components in strict carnivores: A comparative study with cheetah fecal inoculum.
Depauw, S., G. Bosch, M. Hesta, K. Whitehouse-Tedd, W. H. Hendriks, J. Kaandorp, and G. P. J. Janssens. 2012.
J. Anim. Sci. 90:2540-2548. doi:10.2527/jas.2011-4377
“End-product profile per unit of OM differed among substrates (Table 3). The greatest total SCFA production was recorded for FOS (P < 0.05), followed by collagen, casein, and glucosamine (P < 0.05). The FOS and collagen showed comparable acetate production. Collagen not only had a high production of total SCFA but also resulted in a greater acetate to propionate ratio relative to all other substrates (8.41:1 for collagen and 1.67:1–2.97:1 for other substrates). Chicken cartilage and glucosamine-chondroitin produced similar total SCFA production, which was moderate compared with FOS (P < 0.05). Total SCFA production from incubated rabbit bone and skin was low (P < 0.05), whereas total SCFA production from rabbit hair was negligible and comparable with the negative control cellulose. Butyrate production was greatest for casein and glucosamine (P < 0.05). Incubation with casein resulted in the greatest total BCFA production (P < 0.05), which was more than double compared with all other substrates that had similar total BCFA production. Considerable variation in BCFA ratios was observed among substrates. In all animal substrates, isovalerate was the main BCFA, whereas fermentation of FOS, glucosamine, and glucosamine-chondroitin led to valerate as the main BCFA. The greatest amount of ammonia production was observed for casein, collagen, and rabbit bone (P < 0.05), whereas the least ammonia production was detected for FOS, cellulose, and rabbit hair (P < 0.05).”
[Gam2012] Evidence type: non-human animal and human cell in vitro experiments
GPR109A as an Anti-Inflammatory Receptor in Retinal Pigment Epithelial Cells and Its Relevance to Diabetic Retinopathy.
Gambhir D, Ananth S, Veeranan-Karmegam R, et al.
Investigative Ophthalmology & Visual Science. 2012;53(4):2208-2217. doi:10.1167/iovs.11-8447.
“GPR109A is the G-protein–coupled receptor responsible for mediating the antilipolytic actions of niacin (nicotinic acid), a B-complex vitamin and also a drug used widely to lower blood lipid levels.1 β-hydroxybutyrate (β-HB) is the physiologic ligand for this receptor.2 GPR109A expression was initially thought to be limited to adipocytes, the cell type in which its antilipolytic functions are most warranted, and immune cells.3–5 Recent reports, however, have described expression of the receptor in a number of other cell types, including hepatocytes6 and epithelial cells of the small intestine and colon.7,8 In addition, we demonstrated GPR109A expression in the retinal pigment epithelium (RPE), localized specifically to the basolateral membrane.9 Although GPR109A is most noted functionally for its antilipolytic effects in adipocytes, recent studies suggest that activation of the receptor also is associated with novel immunomodulatory responses.10–12 We have characterized expression of GPR109A in RPE; however, the functional significance of receptor expression in this cell type remains unknown.”
[Gon2016] Evidence type: review
Regulation of colonic epithelial butyrate transport: Focus on colorectal cancer
Pedro Gonçalves and Fátima Martel
Porto Biomedical Journal Volume 1, Issue 3, July–August 2016, Pages 83-91
“The most important molecular mechanisms involved in the anticarcinogenic effect of BT are dependent on its intracellular concentration (because HDAC expression is overregulated,41,42 while BT membrane receptors (GPR109A and GPR43) are silenced or downregulated in CRC34,38). So, knowledge on the mechanisms involved in its membrane transport is relevant to both its physiological and pharmacological benefits. Also, changes in transporter expression or function will have an obvious impact on the effect of BT, and therefore, knowledge on the regulation of its membrane transport seems particularly important.
[…]
“[D]ifferences in MCT1, SMCT1 and BCRP expression between normal colonocytes and tumoral cells contribute to the different effects of BT in these cells (‘the BT paradox’). More specifically, BT is transported into normal colonic epithelial cells by both MCT1 and SMCT1, but its intracellular concentration is kept low because it is efficiently metabolized and effluxed from these cells by BCRP-mediated transport. In contrast, colonic epithelial tumoral cells show a decrease in SMCT1 protein expression, and BT is taken up by these cells through MCT1. In these cells, BT accumulates intracellularly because it is inefficiently metabolized (due to the fact that glucose becomes the primary energy source of these cells) and because there is a reduction in BCRP expression.”
[Hin2002] Evidence type: human cell in vitro experiment
The Effects of Short-Chain Fatty Acids on Human Colon Cancer Cell Phenotype Are Associated with Histone Hyperacetylation
Brian F. Hinnebusch, Shufen Meng, James T. Wu, Sonia Y. Archer, and Richard A. Hodin
J. Nutr. May 1, 2002 vol. 132 no. 5 1012-1017
“The short-chain fatty acid (SCFA) butyrate is produced via anaerobic bacterial fermentation within the colon and is thought to be protective in regard to colon carcinogenesis. Although butyrate (C4) is considered the most potent of the SCFA, a variety of other SCFA also exist in the colonic lumen. Butyrate is thought to exert its cellular effects through the induction of histone hyperacetylation. We sought to determine the effects of a variety of the SCFA on colon carcinoma cell growth, differentiation and apoptosis. HT-29 or HCT-116 (wild-type and p21-deleted) cells were treated with physiologically relevant concentrations of various SCFA, and histone acetylation state was assayed by acid-urea-triton-X gel electrophoresis and immunoblotting. Growth and apoptotic effects were studied by flow cytometry, and differentiation effects were assessed using transient transfections and Northern blotting. Propionate (C3) and valerate (C5) caused growth arrest and differentiation in human colon carcinoma cells. The magnitude of their effects was associated with a lesser degree of histone hyperacetylation compared with butyrate. Acetate (C2) and caproate (C6), in contrast, did not cause histone hyperacetylation and also had no appreciable effects on cell growth or differentiation. SCFA-induced transactivation of the differentiation marker gene, intestinal alkaline phosphatase (IAP), was blocked by histone deacetylase (HDAC), further supporting the critical link between SCFA and histones. Butyrate also significantly increased apoptosis, whereas the other SCFA studied did not. The growth arrest induced by the SCFA was characterized by an increase in the expression of the p21 cell-cycle inhibitor and down-regulation of cyclin B1 (CB1). In p21-deleted HCT-116 colon cancer cells, the SCFA did not alter the rate of proliferation. These data suggest that the antiproliferative, apoptotic and differentiating properties of the various SCFA are linked to the degree of induced histone hyperacetylation. Furthermore, SCFA-mediated growth arrest in colon carcinoma cells requires the p21 gene.”
[Blo2011] Evidence type: in vitro experiments
Butyrate elicits a metabolic switch in human colon cancer cells by targeting the pyruvate dehydrogenase complex.
Blouin, J.-M., Penot, G., Collinet, M., Nacfer, M., Forest, C., Laurent-Puig, P., Coumoul, X., Barouki, R., Benelli, C. and Bortoli, S. (2011)
Int. J. Cancer, 128: 2591–2601. doi:10.1002/ijc.25599
“Butyrate, a short-chain fatty acid produced by the colonic bacterial fermentation is able to induce cell growth inhibition and differentiation in colon cancer cells at least partially through its capacity to inhibit histone deacetylases. Since butyrate is expected to impact cellular metabolic pathways in colon cancer cells, we hypothesize that it could exert its antiproliferative properties by altering cellular metabolism. We show that although Caco2 colon cancer cells oxidized both butyrate and glucose into CO2, they displayed a higher oxidation rate with butyrate as substrate than with glucose. Furthermore, butyrate pretreatment led to an increase cell capacity to oxidize butyrate and a decreased capacity to oxidize glucose, suggesting that colon cancer cells, which are initially highly glycolytic, can switch to a butyrate utilizing phenotype, and preferentially oxidize butyrate instead of glucose as energy source to produce acetyl coA. Butyrate pretreated cells displayed a modulation of glutamine metabolism characterized by an increased incorporation of carbons derived from glutamine into lipids and a reduced lactate production. The butyrate-stimulated glutamine utilization is linked to pyruvate dehydrogenase complex since dichloroacetate reverses this effect. Furthermore, butyrate positively regulates gene expression of pyruvate dehydrogenase kinases and this effect involves a hyperacetylation of histones at PDK4 gene promoter level. Our data suggest that butyrate exerts two distinct effects to ensure the regulation of glutamine metabolism: it provides acetyl coA needed for fatty acid synthesis, and it also plays a role in the control of the expression of genes involved in glucose utilization leading to the inactivation of PDC.”
[Jas1985] Evidence type: armchair
Diet, butyric acid and differentiation of gastrointestinal tract tumours.
Jass JR
Med Hypotheses. 1985 Oct;18(2):113-8.
“Abstract
“Butyric acid has two contrasting functional roles. As a product of fermentation within the human colon, it serves as the most important energy source for normal colorectal epithelium. It also promotes the differentiation of cultured malignant cells. A switch from aerobic to anaerobic metabolism accompanies neoplastic transformation in the colorectum. The separate functional roles for n-butyrate may reflect the different metabolic activities of normal and neoplastic tissues. Relatively low intracolonic levels of n-butyrate are associated with a low fibre diet. Deficiency of n-butyrate, coupled to the increased energy requirements of neoplastic tissues, may promote the switch to anaerobic metabolism. The presence of naturally occurring differentiating agents, such as n-butyrate, may modify the patterns of growth and differentiation of gastrointestinal tumours.”
[Ham2008] Evidence type: review
Review article: the role of butyrate on colonic function.
HAMER, H. M., JONKERS, D., VENEMA, K., VANHOUTVIN, S., TROOST, F. J. and BRUMMER, R.-J. (2008)
Alimentary Pharmacology & Therapeutics, 27: 104–119. doi:10.1111/j.1365-2036.2007.03562.x
“Although some controlled studies with enemas containing butyrate or SCFA mixtures in UC patients did not find beneficial effects121 or only trends towards clinical improvement,46, 118, 119 various other studies revealed a significant improvement in clinical and inflammatory parameters.45, 115, 120, 124, 126 Studies in patients with diversion colitis reported inconsistent results with regard to improvement in clinical symptoms and inflammatory parameters in response to administration of mixtures of SCFAs vs. placebo.96, 114 Two other human intervention studies determined mucosal cell proliferation in patients after Hartmann’s procedure and found trophic effects of SCFA mixtures in the mucosa of the closed rectal and sigmoid segment.73, 116”
“The effects of butyrate containing enemas on radiation proctitis113, 117, 122, 125 and pouchitis123 have been studied in small groups and besides one report125 that showed that butyrate was an effective treatment of radiation proctitis, other studies did not report clear-cut beneficial effects of SCFA irrigation in these two patient groups.”
[Ham2010] Evidence type: human experiment
Effect of butyrate enemas on inflammation and antioxidant status in the colonic mucosa of patients with ulcerative colitis in remission.
Hamer HM, Jonkers DM, Vanhoutvin SA, Troost FJ, Rijkers G, de Bruïne A, Bast A, Venema K, Brummer RJ.
Clin Nutr. 2010 Dec;29(6):738-44. doi: 10.1016/j.clnu.2010.04.002. Epub 2010 May 15.
“Abstract
“BACKGROUND & AIMS:
“Butyrate, produced by colonic fermentation of dietary fibers is often hypothesized to beneficially affect colonic health. This study aims to assess the effects of butyrate on inflammation and oxidative stress in subjects with chronically mildly elevated parameters of inflammation and oxidative stress.
“METHODS:
“Thirty-five patients with ulcerative colitis in clinical remission daily administered 60 ml rectal enemas containing 100mM sodium butyrate (n=17) or saline (n=18) during 20 days (NCT00696098). Before and after the intervention feces, blood and colonic mucosal biopsies were obtained. Parameters of antioxidant defense and oxidative damage, myeloperoxidase, several cytokines, fecal calprotectin and CRP were determined.
“RESULTS:
“Butyrate enemas induced minor effects on colonic inflammation and oxidative stress. Only a significant increase of the colonic IL-10/IL-12 ratio was found within butyrate-treated patients (p=0.02), and colonic concentrations of CCL5 were increased after butyrate compared to placebo treatment (p=0.03). Although in general butyrate did not affect colonic glutathione levels, the effects of butyrate enemas on total colonic glutathione appeared to be dependent on the level of inflammation.
“CONCLUSION:
“Although UC patients in remission were characterized by low-grade oxidative stress and inflammation, rectal butyrate enemas showed only minor effects on inflammatory and oxidative stress parameters.”
[Kap2016] Evidence type: review
Fiber and the Risk of Flaring in Patients With Inflammatory Bowel Diseases: Lessons From the Crohn’s and Colitis Foundation of America Database
Gilaad G. Kaplan, MD, MPH, FRCPC
Clinical Gastroenterology and Hepatology , Volume 14 , Issue 8 , 1137 – 1139
“After reviewing the study from Brotherton et al and prior literature, information for patients with IBD on the effects of fiber on the risk of flaring is unclear. The current article adds to this discussion but does not definitively answer the question. Overall, the data suggest that in the absence of a known fibrostenotic stricture with obstructive symptoms, a high fiber diet is likely safe in patients with IBD and may impart a weak benefit. Yet, answering these clinically relevant questions with more confidence and detail is within our grasp. The advent of e-cohorts offers the potential to transform research in the future by allowing investigators to design cost-efficient Web-based clinical studies, particularly for interventional environmental clinical trials.”
[Kor1990] Evidence type: non-human animal experiment
Parenteral nutrition supplemented with short-chain fatty acids: effect on the small-bowel mucosa in normal rats.
Koruda MJ1, Rolandelli RH, Bliss DZ, Hastings J, Rombeau JL, Settle RG.
Am J Clin Nutr. 1990 Apr;51(4):685-9.
“Abstract
“When enteral nutrition is excluded from animals maintained solely with total parenteral nutrition (TPN), atrophy of the intestinal mucosa is observed. Short-chain fatty acids (SCFAs) are produced in the colon by the fermentation of dietary carbohydrates and fiber polysaccharides and have been shown to stimulate mucosal-cell mitotic activity in the intestine. This study compared the effects of an intravenous and an intracecal infusion of SCFAs on the small-bowel mucosa. Rats received standard TPN, TPN with SCFAs (sodium acetate, propionate, and butyrate), TPN with an intracecal infusion of SCFAs, or rat food. After 7 d jejunal and ileal mucosal weights, DNA, RNA, and protein were determined. Standard TPN produced significant atrophy of the jejunal and ileal mucosa. Both the intracecal and intravenous infusion of SCFAs significantly reduced the mucosal atrophy associated with TPN. The intravenous and intracolonic infusion of SCFAs were equally effective in inhibiting small-bowel mucosal atrophy.”
[Mal2015] Evidence type: non-human animal experiment
Intraperitoneal administration of butyrate prevents the severity of acetic acid colitis in rats
Joshua J. Malago and Catherine L. Sangu
Zhejiang Univ Sci B. 2015 Mar; 16(3): 224–234. doi: 10.1631/jzus.B1400191
“Earlier studies that linked the development of UC and butyrate levels in the colon, observed that deficiency of butyrate leads to disease development and that restoration of butyrate levels by intracolonic infusion treats UC (Roediger, 1980). Since then, butyrate enemas have popularly been used as medicaments stemming from their potential to impart beneficial attributes to the colon. This potential involves an increase in mechanical strength of injured colonic mucosa to hasten the healing process (Bloemen et al., 2010; Mathew et al., 2010), suppression of IL-8 production by intestinal epithelial cells to protect against the inflammatory process (Malago et al., 2005), and clinical remission of UC by protecting against inflammatory and oxidative stress parameters of the disease (Hamer et al., 2010b). Much as butyrate tends to impart a protective effect, several authors have indicated failures or limited success of butyrate to relieve IBD patients (Harig et al., 1989; Sanderson, 1997; Hamer et al., 2010b).”
…
“Topical administration of butyrate to cure colitis has been fairly well demonstrated (Scheppach et al., 1992; Hamer et al., 2010a; 2010b). This is done mainly through intrarectal administration of enemas that contain butyrate. The procedure is one of the earliest approaches to treat UC even in patients who had been unresponsive to or intolerant of standard therapy (Scheppach et al., 1992). The intrarectally administered butyrate needs to be absorbed before it works. Normally butyrate absorption mainly occurs in proximal colon whose function is impaired during UC. This hinders absorption of topically administered butyrate and may not benefit UC patients. However, butyrate absorption in the colon can be increased by manipulating electrolyte composition in the rectal lumen (Holtug et al., 1995) since rectal butyrate absorption remains normal during UC (Hove et al., 1995). Thus, topical butyrate, given intrarectally in form of SB, plays a double role; firstly by employing sodium ions, it accelerates rectal absorption of SB and secondly, the absorbed butyrate imparts healing to the colonocytes. The end result is epithelial proliferation to restore the damaged epithelium, especially the lost colonic epithelial continuity.”
…
“We have demonstrated the potential of intraperitoneally administered butyrate to prevent the severity of AA-induced UC lesions. To the best of our knowledge, this finding has not been reported before. However, the systemic effect of butyrate to other body systems and organs has been reported. For instance, intraperitoneal injection of butyrate at 50–200 mg/kg body weight decreases gentamicin-induced nephrotoxicity in rats by enhancing renal antioxidant enzyme activity and expression of prohibitin protein (Sun et al., 2013). When given at 1200 mg/kg, intraperitoneal butyrate ameliorates an aging-associated deficit in object recognition memory in rats (Reolon et al., 2011). Silingardi et al. (2010) further demonstrated that chronic intraperitoneal administration of butyrate to long-term monocularly deprived adult rats causes a complete recovery of visual acuity. A more recent study has also reported that intraperitoneal injections of butyrate for 28 d to adult C57BL/6 mice prevent repressed contextual fear memory caused by isoflurane (Zhong et al., 2014). All these facts and our own study affirm that butyrate has a potential to impart protective roles to various body organs and systems through systemic administration.”
[Mil2017] Evidence type: non-human animal experiment
Supplementation of Low- and High-fat Diets with Fermentable Fiber Exacerbates Severity of DSS-induced Acute Colitis.
Miles JP, Zou J, Kumar MV, Pellizzon M, Ulman E, Ricci M, Gewirtz AT, Chassaing B.
“Abstract
“BACKGROUND:
“Lack of dietary fiber has been suggested to increase the risk of developing various chronic inflammatory diseases, whereas supplementation of diets with fiber might offer an array of health-promoting benefits. Consistent with this theme, we recently reported that in mice, compositionally defined diets that are made with purified ingredients and lack fermentable fiber promote low-grade inflammation and metabolic syndrome, both of which could be ameliorated by supplementation of such diets with the fermentable fiber inulin.
“METHODS:
“Herein, we examined if, relative to a grain-based mouse diet (chow), compositionally defined diet consumption would impact development of intestinal inflammation induced by dextran sulfate sodium (DSS) and moreover, whether DSS-induced colitis might also be attenuated by diets supplemented with inulin.
“RESULTS:
“Analogous to their promotion of low-grade inflammation, compositionally defined diet of high- and low-fat content with cellulose increased the severity of DSS-induced colitis relative to chow. However, in contrast to the case of low-grade inflammation, addition of inulin, but not the insoluble fiber cellulose, further exacerbated the severity of colitis and its associated clinical manifestations (weight loss and bleeding) in both low- and high-fat diets.
“CONCLUSIONS:
“While inulin, and perhaps other fermentable fibers, can ameliorate low-grade inflammation and associated metabolic disease, it also has the potential to exacerbate disease severity in response to inducers of acute colitis.”
[Miz2011] Evidence type: review
Autophagy: renovation of cells and tissues.
Mizushima N, Komatsu M.
Cell. 2011 Nov 11;147(4):728-41. doi: 10.1016/j.cell.2011.10.026.
“Autophagy is the major intracellular degradation system by which cytoplasmic materials are delivered to and degraded in the lysosome. However, the purpose of autophagy is not the simple elimination of materials, but instead, autophagy serves as a dynamic recycling system that produces new building blocks and energy for cellular renovation and homeostasis. Here we provide a multidisciplinary review of our current understanding of autophagy’s role in metabolic adaptation, intracellular quality control, and renovation during development and differentiation. We also explore how recent mouse models in combination with advances in human genetics are providing key insights into how the impairment or activation of autophagy contributes to pathogenesis of diverse diseases, from neurodegenerative diseases such as Parkinson disease to inflammatory disorders such as Crohn disease.”
[Nei2015] Evidence type: review
The Role of Microbial Amino Acid Metabolism in Host Metabolism.
Neis EPJG, Dejong CHC, Rensen SS.
Nutrients. 2015;7(4):2930-2946. doi:10.3390/nu7042930.
“Although protein breakdown followed by amino acid absorption in the small intestine is a rather efficient process, substantial amounts of amino acids seem to escape assimilation in the small intestine in humans [38]. These amino acids can subsequently be used by the microbiota in the colon, or transported from the lumen into the portal blood stream. In addition, the host itself produces substrates such as glycoproteins (e.g., mucins) which contribute to the available amino acids within the colon [39]. ”
[…]
“Regarding the large intestine, it appears that amino acids are not significantly absorbed by the colonic mucosa, but rather are intensively metabolized by the large intestinal microbiota [23]. This higher rate of bacterial protein fermentation has been related to high pH and low carbohydrate availability in the large intestine [22]. The preferred amino acid substrates of colonic bacteria include lysine, arginine, glycine, and the BCAA leucine, valine, and isoleucine [32], resulting in the generation of a complex mixture of metabolic end products including among others ammonia, SCFA (acetate, propionate, and butyrate), and branched-chain fatty acids (BCFA; valerate, isobutyrate, and isovalerate). “
[Pen2007] Evidence type: non-human animal experiment
Effects of Butyrate on Intestinal Barrier Function in a Caco-2 Cell Monolayer Model of Intestinal Barrier
Luying Peng, Zhenjuan He, Wei Chen, Ian R Holzman and Jing Lin
Pediatric Research (2007) 61, 37–41; doi:10.1203/01.pdr.0000250014.92242.f3
“In premature infants, the maturation of the intestinal barrier function does not develop properly in the absence of enteral nutrients (6). Intestinal barrier function is significantly less developed in full-term newborn piglets receiving total parental nutrition compared with those receiving enteral nutrition (7). Production of SCFA in the bowel may be crucial for gastrointestinal adaptation and maturation in the early stage of postnatal life (8). However, overproduction and/or accumulation of SCFA in the bowel due to maldigestion and bacterial overgrowth may be toxic to mucosal cells and cause intestinal mucosal injury (9,10). Overproduction and/or accumulation of SCFA in the bowel and inability to clear the intraluminal SCFA because of poor gastrointestinal motility in premature infants have been hypothesized to play a role in the pathogenesis of neonatal NEC (11).”
[Rah2014] Evidence type: non-human animal experiment
The β-hydroxybutyrate receptor HCA2 activates a neuroprotective subset of macrophages.
Rahman M, Muhammad S, Khan MA, Chen H, Ridder DA, Müller-Fielitz H, Pokorná B, Vollbrandt T, Stölting I, Nadrowitz R, Okun JG, Offermanns S, Schwaninger M.
Nat Commun. 2014 May 21;5:3944. doi: 10.1038/ncomms4944.
“Abstract
“The ketone body β-hydroxybutyrate (BHB) is an endogenous factor protecting against stroke and neurodegenerative diseases, but its mode of action is unclear. Here we show in a stroke model that the hydroxy-carboxylic acid receptor 2 (HCA2, GPR109A) is required for the neuroprotective effect of BHB and a ketogenic diet, as this effect is lost in Hca2(-/-) mice. We further demonstrate that nicotinic acid, a clinically used HCA2 agonist, reduces infarct size via a HCA2-mediated mechanism, and that noninflammatory Ly-6C(Lo) monocytes and/or macrophages infiltrating the ischemic brain also express HCA2. Using cell ablation and chimeric mice, we demonstrate that HCA2 on monocytes and/or macrophages is required for the protective effect of nicotinic acid. The activation of HCA2 induces a neuroprotective phenotype of monocytes and/or macrophages that depends on PGD2 production by COX1 and the haematopoietic PGD2 synthase. Our data suggest that HCA2 activation by dietary or pharmacological means instructs Ly-6C(Lo) monocytes and/or macrophages to deliver a neuroprotective signal to the brain.”
[Ras1988] Evidence type: in vitro experiment
Degradation of amino acids to short-chain fatty acids in humans. An in vitro study.
Rasmussen HS, Holtug K, Mortensen PB.
Scand J Gastroenterol. 1988 Mar;23(2):178-82.
“Short-chain fatty acids (SCFA) originate mainly in the colon through bacterial fermentation of polysaccharides. To test the hypothesis that SCFA may originate from polypeptides as well, the production of these acids from albumin and specific amino acids was examined in a faecal incubation system. Albumin was converted to all C2-C5-fatty acids, whereas amino acids generally were converted to specific SCFA, most often through the combination of a deamination and decarboxylation of the amino acids, although more complex processes also took place. This study indicates that a part of the intestinal SCFA may originate from polypeptides, which apparently are the major source of those SCFA (isobutyrate, valerate, and isovalerate) only found in small amounts in the healthy colon. Moreover, gastrointestinal disease resulting in increased proteinous material in the colon (exudation, mucosal desquamation, bleeding, and so forth) may hypothetically influence SCFA production.”
[Roe1980]
Evidence type: human experiment
[Roe1980] (1, 2) Evidence type: human experiment
Roediger WE.
Lancet. 1980 Oct 4;2(8197):712-5.
“The view that UC might be due to a metabolic defect in the epithelial cells[5,6] has received little general recognition. The present study was undertaken to assess the metabolic performance of the mucosa in UC and especially to explore whether a metabolic abnormality could be detected. To facilitate this approach a method of preparing suspensions of colonocytes was devised.[7] Colonocytes have been used to determine the utilisation of respiratory fuels by the non-diseased ascending and descending colon in man.[8] The results showed that short-chain fatty acid (SCFA), especially n-butyrate of bacterial origin, was the predominant contributor to cellular oxidation and that a large proportion of the carbon atoms of colonocyte respiration was derived from SCFAs. Mucosa of the distal colon depended metabolically mostly on n-butyrate, whereas the proximal colonic mucosa depended mostly on glucose and glutamine for respiratory fuel.[8] These same respiratory fuels were chosen for the investigation of colonocytes prepared from the mucosa of patients with ulcerative colitis.
…
“Generation of 14C02 from radioactively labelled butyrate was observed for at least 40 min. Production of 14C02 was linear whenever this could be tested for 60 min. Generation of 14C02 was significantly less in quiescent and acute-colitis cells than in controls (p = <0.001) (table II). Some of the oxidised butyrate appeared as ketone bodies (acetoacetate and β-hydroxybutyrate, table III). The diminished production of ketone bodies mirrors the decreased oxidation of butyrate to CO2. Ketogenesis was significantly lower in the quiescent-colitis group than the control group and lower still in the acute-colitis group.
…
“The metabolism of colonocytes from patients with UC seemed to differ in three respects from the metabolism of colonocytes prepared from non-ulcerated and apparently normal mucosa. In UC: 1. Butyrate oxidation to C02 and ketone bodies was significantly impaired, and the impairment correlated with the acute or chronic involvement of the mucosa. 2. Glucose oxidation was increased. 3. Glutamine oxidation was increased.”
…
“Ketogenesis was significantly lower in the quiescent-colitis group than the control group and lower still in the acute-colitis group.”
[Roe1993] Evidence type: non-human animal experiment
Sulphide impairment of substrate oxidation in rat colonocytes: a biochemical basis for ulcerative colitis?
Roediger WE, Duncan A, Kapaniris O, Millard S.
Clin Sci (Lond). 1993 Nov;85(5):623-7.
“Abstract
“Isolated colonic epithelial cells of the rat were incubated for 40 min with [6-14C]glucose and n-[1-14C]butyrate in the presence of 0.1-2.0 mmol/l NaHS, a concentration range found in the human colon. Metabolic products, 14CO2, acetoacetate, beta-hydroxybutyrate and lactate, were measured and injury to cells was judged by diminished production of metabolites. 2. Oxidation of n-butyrate to CO2 and acetoacetate was reduced at 0.1 and 0.5 mmol/l NaHS, whereas glucose oxidation remained unimpaired. At 1.0-2.0 mmol/l NaHS, n-butyrate and glucose oxidation were dose-dependently reduced at the same rate. 3. To bypass short-chain acyl-CoA dehydrogenase activity necessary for butyrate oxidation, ketogenesis from crotonate was measured in the presence of 1.0 mmol/l NaHS. Suppression by sulphide of ketogenesis from crotonate (-10.5 +/- 6.1%) compared with control conditions was not significant, whereas suppression of ketogenesis from n-butyrate (-36.00 +/- 5.14%) was significant (P = < 0.01). Inhibition of FAD-linked oxidation was more affected by NaHS than was NAD-linked oxidation. 4. L-Methionine (5.0 mmol/l) significantly redressed the impaired beta-oxidation induced by NaHS. Methionine equally improved CO2 and ketone body production, suggesting a global reversal of the action of sulphide. 5. Sulphide-induced oxidative changes closely mirror the impairment of beta-oxidation observed in colonocytes of patients with ulcerative colitis. A hypothesis for the disease process of ulcerative colitis is that sulphides may form persulphides with butyryl-CoA, which would inhibit cellular short-chain acyl-CoA deHydrogenase and beta-oxidation to induce an energy-deficiency state in colonocytes and mucosal inflammation.”
[Rol1997] Evidence type: non-human animal experiment
Intravenous butyrate and healing of colonic anastomoses in the rat.
Rolandelli RH, Buckmire MA, Bernstein KA.
Dis Colon Rectum. 1997 Jan;40(1):67-70.
“PURPOSE:
“Intracolonic infusions of short chain fatty acids promote healing of colonic anastomoses. Because the intravenous route may have wider clinical application, we studied the effect of intravenous n-butyrate on the mechanical strength of colonic anastomoses in the rat.
“METHODS:
“After placement of an indwelling intravenous catheter, the descending colon was transected and an anastomosis was performed. Rats were then randomized to receive total parenteral nutrition (TPN group; n = 15) or total parenteral nutrition plus 130 mM/l of n-butyrate (TPN+BUT group; n = 13). On the fifth postoperative day, bursting pressure and bowel wall tension of the anastomoses were measured in situ. Anastomotic tissues were analyzed for hydroxyproline.
“RESULTS:
“The TPN+BUT group had a significantly higher bursting pressure (107.5 +/- 30.3 vs. 83 +/- 41.0 mmHg; P = 0.04) and bowel wall tension (20.7 +/- 7.6 vs. 14.1 +/- 9.9 Newton; P = 0.03). Tissue hydroxyproline was not different between the two groups (TPN, 45.8 +/- 9.2, and TPN+BUT, 47.9 +/- 2.9 microg/mg tissue nitrogen).
“CONCLUSIONS:
“We conclude that intravenous butyrate improves mechanical strength of a colonic anastomosis without a detectable change in total collagen content.”
[Rom1990] Evidence type: review
Short-Chain Fatty Acids.
Rombeau J.L., Kripke S.A., Settle R.G. (1990)
In: Kritchevsky D., Bonfield C., Anderson J.W. (eds) Dietary Fiber. Springer, Boston, MA
“As mentioned previously hepatic metabolism of butyrate and acetate results in the production of glutamine and the ketone bodies acetoacetate and which are the preferred oxidative fuels of enterocytes (Windmueller and Spaeth, 1978). The enteral or parenteral provision of glutamine and acetoacetate has been shown to be trophic to both small and large intestinal mucosa (Fox et al., 1987; Kripke et al., 1988a).”
[Sen2006] Evidence type: review
Does butyrate protect from colorectal cancer?
Sengupta S, Muir JG, Gibson PR.
J Gastroenterol Hepatol. 2006 Jan;21(1 Pt 2):209-18.
“Abstract
“Butyrate, the four-carbon fatty acid, is formed in the human colon by bacterial fermentation of carbohydrates (including dietary fiber), and putatively suppresses colorectal cancer (CRC). Butyrate has diverse and apparently paradoxical effects on cellular proliferation, apoptosis and differentiation that may be either pro-neoplastic or anti-neoplastic, depending upon factors such as the level of exposure, availability of other metabolic substrate and the intracellular milieu. In humans, the relationship between luminal butyrate exposure and CRC has been examined only indirectly in case-control studies, by measuring fecal butyrate concentrations, although this may not accurately reflect effective butyrate exposure during carcinogenesis. Perhaps not surprisingly, results of these investigations have been mutually contradictory. The direct effect of butyrate on tumorigenesis has been assessed in a number of in vivo animal models, which have also yielded conflicting results. In part, this may be explained by methodological differences in the amount and route of butyrate administration, which are likely to significantly influence delivery of butyrate to the distal colon. Nonetheless, there appears to be some evidence that delivery of an adequate amount of butyrate to the appropriate site protects against early tumorigenic events. Future study of the relationship between butyrate and CRC in humans needs to focus on risk stratification and the development of feasible strategies for butyrate delivery.”
[Shi2013] Evidence type: non-human animal experiment
Suppression of Oxidative Stress by β-Hydroxybutyrate, an Endogenous Histone Deacetylase Inhibitor.
Shimazu T, Hirschey MD, Newman J, et al.
Science (New York, NY). 2013;339(6116):211-214. doi:10.1126/science.1227166.
“Concentrations of acetyl–coenzyme A and nicotinamide adenine dinucleotide (NAD+) affect histone acetylation and thereby couple cellular metabolic status and transcriptional regulation. We report that the ketone body d-β-hydroxybutyrate (βOHB) is an endogenous and specific inhibitor of class I histone deacetylases (HDACs). Administration of exogenous βOHB, or fasting or calorie restriction, two conditions associated with increased βOHB abundance, all increased global histone acetylation in mouse tissues. Inhibition of HDAC by βOHB was correlated with global changes in transcription, including that of the genes encoding oxidative stress resistance factors FOXO3A and MT2. Treatment of cells with βOHB increased histone acetylation at the Foxo3a and Mt2 promoters, and both genes were activated by selective depletion of HDAC1 and HDAC2. Consistent with increased FOXO3A and MT2 activity, treatment of mice with βOHB conferred substantial protection against oxidative stress.”
[Sin2014] Evidence type: non-human animal experiment
Activation of Gpr109a, Receptor for Niacin and the Commensal Metabolite Butyrate, Suppresses Colonic Inflammation and Carcinogenesis
Singh, Nagendra et al.
Immunity , Volume 40 , Issue 1 , 128 – 139
“The most widely studied function of butyrate is its ability to inhibit histone deacetylases. However, cell surface receptors have been identified for butyrate; these receptors, GPR43 and GPR109A (also known as hydroxycarboxylic acid receptor 2 or HCA2), are G protein coupled and are expressed in colonic epithelium, adipose tissue, and immune cells (Blad et al., 2012, Ganapathy et al., 2013). GPR43-deficient mice undergo severe colonic inflammation and colitis in DSS-induced colitis model and the GPR43 agonist acetate protects germ-free mice from DSS-induced colitis (Maslowski et al., 2009). Although GPR43 is activated by all three SCFAs, GPR109A (encoded by Niacr1) is activated only by butyrate (Blad et al., 2012, Taggart et al., 2005). GPR109A is also activated by niacin (vitamin B3) (Blad et al., 2012, Ganapathy et al., 2013). In colonic lumen, butyrate is generated at high concentrations (10–20 mM) by gut microbiota and serves as an endogenous agonist for GPR109A (Thangaraju et al., 2009). We have shown that Gpr109a expression in colon is induced by gut microbiota and is downregulated in colon cancer (Cresci et al., 2010, Thangaraju et al., 2009). Gpr109a in immune cells plays a nonredundant function in niacin-mediated suppression of inflammation and atherosclerosis (Lukasova et al., 2011). Gut microbiota also produce niacin. Niacin deficiency in humans results in pellagra, characterized by intestinal inflammation, diarrhea, dermatitis, and dementia (Hegyi et al., 2004). It is of great clinical relevance that lower abundance of GPR109A ligands niacin and butyrate in gut is associated with colonic inflammation.”
[…]
“Activation of Gpr109a Suppresses Colonic Inflammation and Carcinogenesis in the Absence of Gut Microbiota or Dietary Fiber
“We then examined the relevance of niacin, a pharmacologic agonist for GPR109A, to colonic inflammation. For this, we first depleted gut microbiota with antibiotics, which reduces the production of butyrate, the endogenous agonist for GPR109A. Antibiotic treatment resulted in >300-fold reduction in aerobic and anaerobic bacterial counts in the stool (data not shown). Antibiotic treatment increased DSS-induced weight loss, diarrhea, and bleeding in WT mice (Figures 7B and S6A). Consistent with increased inflammation, we found that antibiotic treatment increased the number of polyps (8.2 ± 2.2 polyps/mouse with antibiotics; 1.6 ± 1.5 polyps/mouse without antibiotics) in WT mice (Figures 7C and 7D). We then tested whether administration of niacin protects antibiotic-treated mice against colonic inflammation and carcinogenesis. Niacin was added to drinking water along with antibiotic cocktail. Niacin ameliorated AOM+DSS-induced weight loss, diarrhea, and bleeding and reduced colon cancer development in antibiotic-treated WT mice (Figures 7B–7D and S6A). Consistent with a role of niacin in IL-18 induction, the protective effect of niacin in DSS-induced weight loss and diarrhea in antibiotic-treated Il18−/− mice was significantly blunted (Figure S6B). Niacin did not alter the development of weight loss, diarrhea, rectal bleeding, and colon cancer in antibiotic-treated Niacr1−/− mice, suggesting an essential role of Gpr109a in niacin-mediated promotion of colonic health (Figures 7B–7D and S6A). Antibiotic treatment reduced colonic inflammation and number of polyps in Niacr1−/− mice. This may be due to the presence of altered colitogenic gut microbiota in Niacr1−/− animals. ”
[…]
“Although it has been known for decades that the commensal metabolite butyrate suppresses inflammation and carcinogenesis in colon, the exact identity of molecular target(s) of butyrate in this process remained elusive. The present studies identify Gpr109a as an important mediator of butyrate effects in colon and also as a critical molecular link between colonic bacteria and dietary fiber and the host. These findings have important implications for prevention as well as treatment of inflammatory bowel disease and colon cancer and suggest that under conditions of reduced dietary fiber intake and/or decreased butyrate production in colon, pharmacological doses of niacin might be effective to maintain GPR109A signaling and consequently protect colon against inflammation and carcinogenesis.”
[Siv2017] Evidence type: review
Cell-Surface and Nuclear Receptors in the Colon as Targets for Bacterial Metabolites and Its Relevance to Colon Health. <http://europepmc.org/articles/pmc5579649#B58-nutrients-09-00856>-
Sathish Sivaprakasam, Yangzom D. Bhutia, Sabarish Ramachandran, and Vadivel Ganapathy
Nutrients. 2017 August; 9(8): 856.
“As the cell-surface receptors for SCFAs are located on the lumen-facing apical membrane of colonic epithelial cells (see below), the luminal concentrations of these agonists are physiologically relevant. SCFAs are low-affinity agonists for these receptors, and the normal luminal concentrations of these bacterial metabolites are in the millimolar levels, sufficient to activate these receptors from the luminal side. However, some of the molecular targets for these metabolites are either inside the cells (e.g., HDACs) or on the surface of the immune cells located in the lamina propria. Therefore, concentrations of these metabolites inside the colonic epithelial cells and in the lamina propria are relevant to impact these molecular targets. The intracellular target HDAC is inhibited by butyrate and propionate at low micromolar concentrations. There are effective transport systems for SCFAs in the apical membrane of colonic epithelial cells (e.g., proton-coupled and sodium-coupled monocarboxylate transporters) [47], thus making it very likely for these SCFAs to reach intracellular levels sufficient to inhibit HDACs. Even though the luminal concentrations of SCFAs are in the millimolar range, it is unlikely that they reach lamina propria at significant levels to activate the cell-surface receptors present on the mucosal immune cells. These metabolites are present only at micromolar levels in the portal blood [57], indicating that they undergo robust metabolism inside the colonic epithelial cells. This raises the question as to the physiological relevance of these bacterial metabolites to the activation of the cell-surface SCFA receptors in immune cells located in the lamina propria. With regard to this issue, it is important to note that colonic epithelial cells are highly ketogenic; they use acetate and butyrate to generate the ketone body β-hydroxybutyrate [58]. This ketone body is released from the cells into portal blood. As β-hydroxybutyrate is 3–4 times more potent than butyrate in activating its receptor GPR109A, it can be speculated that the colon-derived ketone body is most likely involved in the activation of the SCFA receptor in mucosal immune cells.”
[Tag2005] Evidence type: in vitro non-human animal experiment
(D)-beta-Hydroxybutyrate inhibits adipocyte lipolysis via the nicotinic acid receptor PUMA-G.
Taggart AK1, Kero J, Gan X, Cai TQ, Cheng K, Ippolito M, Ren N, Kaplan R, Wu K, Wu TJ, Jin L, Liaw C, Chen R, Richman J, Connolly D, Offermanns S, Wright SD, Waters MG.
J Biol Chem. 2005 Jul 22;280(29):26649-52. Epub 2005 Jun 1.
“Here we show that the fatty acid-derived ketone body (d)-β-hydroxybutyrate ((d)-β-OHB) specifically activates PUMA-G/HM74a at concentrations observed in serum during fasting. Like nicotinic acid, (d)-β-OHB inhibits mouse adipocyte lipolysis in a PUMA-G-dependent manner and is thus the first endogenous ligand described for this orphan receptor. These findings suggests a homeostatic mechanism for surviving starvation in which (d)-β-OHB negatively regulates its own production, thereby preventing ketoacidosis and promoting efficient use of fat stores.”
[Tot2016] Evidence type: human case study
Crohn’s disease successfully treated with the paleolithic ketogenic diet
Tóth C, Dabóczi A, Howard M, Miller NJ, Clemens Z.
Int J Case Rep Images 2016;7(10):570–578.
“Given the ineffectiveness of standard therapies the parents of the child were seeking for alternative options. When we first met the patient he reported bilateral pain and swelling of the knee, frequent episodes of fever and night sweats as well as fatigue. He looked pale. We offered the paleolithic ketogenic diet along with close monitoring of the patient. The patient started the diet on 4 January 2015. The diet is consisting of animal fat, meat, offal and eggs with an approximate 2:1 fat : protein ratio. Red and fat meats instead of poultry as well as regular intake of organ meats from pork and cattle were encouraged. Grains, milk, dairy, refined sugars, vegetable oils, oilseeds, nightshades and artificial sweeteners were excluded. Small amount of honey was allowed for sweetening. The patient was not taking any supplements. Regular home monitoring of urinary ketones indicated sustained ketosis. Regular laboratory follow-up was used to monitor the course of the disease as well as for giving feedback how to fine tune the diet. The patient was under our close control and gave frequent feedbacks and so we could assess the level of dietary compliance. The patient maintained a high level dietary adherence on the long-term, yet on his birthday, he made a mistake: he has eaten two pieces of commercially available “paleo” cake which contained coconut oil, flour from oilseeds as well as sugar alcohol. Clinical consequences are discussed later. From July 2015 onwards he also consumed small amounts of vegetables and fruits. Given the persistence of certain alterations in laboratory values (mild anemia) on 10 November 2015, despite 10 months on the paleolithic ketogenic diet, we suggested to tighten the diet again. From this time on he did neither consume vegetables and fruits nor vegetable oil containing spices such as cumin and cinnamon.
“Discontinuing medication
“Within two weeks after diet onset the patient discontinued azathioprine, the only medicine he was taking at this time. Currently, he is without medicines for 15 months.
“Symptoms
“The frequent night sweats of the patient disappeared within three weeks after diet onset and thus his sleep improved significantly. The knee pains of the patient began to lessen at 4th week on the diet and completely disappeared by the third month. From this time onwords he regularly went to school by bike (20 km daily). He reported restored energy and increased physical and mental fitness. Although during the eight months before diet onset his weight was declining, following diet onset he began to gain weight. At diet onset his weight was 41 kg and was 152 cm tall (BMI = 17.7). At 12 months after diet onset, his height was 160 cm and weighted 50 kg (BMI: 19.5). The change in his height and weight is depicted in Figure 5. At the time of writing the article he is on the diet for 15 months and is free of symptoms as well as side effects.”
[Tur2011] Evidence type: review
Administration of Substances to Laboratory Animals: Routes of Administration and Factors to Consider
Patricia V Turner, Thea Brabb, Cynthia Pekow, and Mary Ann Vasbinder
J Am Assoc Lab Anim Sci. 2011 Sep; 50(5): 600–613.
“Intraperitoneal administration.
“Injection of substances into the peritoneal cavity is a common technique in laboratory rodents but rarely is used in larger mammals and humans. Intraperitoneal injection is used for small species for which intravenous access is challenging and it can be used to administer large volumes of fluid safely (Table 1) or as a repository site for surgical implantation of a preloaded osmotic minipump. Absorption of material delivered intraperitoneally is typically much slower than for intravenous injection. Although intraperitoneal delivery is considered a parenteral route of administration, the pharmacokinetics of substances administered intraperitoneally are more similar to those seen after oral administration, because the primary route of absorption is into the mesenteric vessels, which drain into the portal vein and pass through the liver.74 Therefore substances administered intraperitoneally may undergo hepatic metabolism before reaching the systemic circulation. In addition, a small amount of intraperitoneal injectate may pass directly across the diaphragm through small lacunae and into the thoracic lymph.”
[Vit2014] Experiment type: metagenomic analysis
Revealing the Bacterial Butyrate Synthesis Pathways by Analyzing (Meta)genomic Data
Marius Vital, Adina Chuang Howe, and James M. Tiedje
mBio. 2014 Mar-Apr; 5(2): e00889-14. Published online 2014 April 22. doi: 10.1128/mBio.00889-14
“Diet is a major external force shaping gut communities (33). Good reviews of studies investigating the influence of diet on butyrate-producing bacteria exist (11 and 34) and suggest that plant-derived polysaccharides such as starch and xylan, as well as cross-feeding mechanisms with lactate-producing bacteria, are the main factors governing their growth. Our metagenomic analysis supports the acetyl-CoA pathway as the main pathway for butyrate production in healthy individuals (Fig. 4), implying that a sufficient polysaccharide supply is probably sustaining a well-functioning butyrate-producing community, at least in these North American subjects. However, the detection of additional amino acid-fed pathways, especially the lysine pathway, indicates that proteins could also play an important role in butyrate synthesis and suggests some flexibility of the microbiota to adapt to various nutritional conditions maintaining butyrate synthesis. Whether the prevalence of amino acid-fed pathway is associated with a protein-rich diet still needs to be assessed. It should be noted that those pathways are not restricted to single substrates, as displayed in Fig. 1, i.e., glutarate and lysine, but additional amino acids, such as aspartate, can be converted to butyrate via those routes as well (26). Furthermore, the acetyl-CoA pathway also can be supplied with substrates derived from proteins either by cross-feeding with the lysine pathway (as discussed above) or by direct fermentation of amino acids to acetyl-CoA (35). However, whereas diet-derived proteins are probably important for butyrate synthesis in the ileum, where epithelial cells use butyrate as a main energy source as well (36), it still needs to be assessed whether enough proteins reach the human colon to serve as a major nutrient source for microorganisms. Another possible colonic protein source could originate with lysed bacterial cells. Enormous viral loads have been detected in this environment, suggesting fast cell/nutrient turnover, which might explain the presence of corresponding pathways in both fecal isolates and metagenomic data (Fig. 1, 4, and 5). Detailed investigations of butyrate-producing communities in the colon of carnivorous animals will add additional key information on the role of proteins in butyrate production in that environment. It should be noted that diet provides only a part of the energy/carbon sources for microbial growth in the colon, since host-derived mucus glycans serve as an important nutrient source as well. Several butyrate-producing organisms do specifically colonize mucus (37), and for some, growth on mucus-derived substrates was shown (38). “
[Wie2017] Evidence type: review
Amino Acid Absorption in the Large Intestine of Humans and Porcine Models.
van der Wielen N, Moughan PJ, Mensink M.
J Nutr. 2017 Aug;147(8):1493-1498. doi: 10.3945/jn.117.248187. Epub 2017 Jun 14.
“Protein digestion and fermentation in the large intestine. Intact proteins that escape the small intestine or produced in the large intestine (mucus, cells, microbial proteins) are digested further in the large intestine by bacterial enzymes and the surviving pancreatic proteases and peptidases (35, 36). This protein degradation has been reported to be highest in the distal large intestine and is m ost likely related to the pH in the different regions (37). The di gested proteins can be used by the microbiota, which produce several metabolites such as SCFAs, ammonia, and amines. These metabolites may be linked to several health outcomes (38).”
[…]
“The large intestine is important for whole-body protein and nitrogen metabolism, in particular via bacterial metabolism. Both small and large intestinal microbiota are capable of synthesizing AAs, and absorption of microbial AAs has been demonstrated to take place in the intestine.”