Similarities between germ-free mice and ketogenic humans
Tracing a chain of ideas
Sometimes the assumptions that scientists start with about what is “good”, “healthy”, or “normal” can cause them to interpret results in a completely different way than someone starting with different assumptions would have. Then, the resulting conclusions become the assumptions in the next round of interpretation, leading to a chain of logic in which one questionable assumption leads to another.
We recently read a paper in which the authors made a series of logical steps, and it became almost comical to us how at each step we would have interpreted their results in an opposite way than they did.
When the results of their experiment are looked at from our perspective, it suggests an intriguing hypothesis:
Maybe some of the health benefits a ketogenic diet are due, not just to the diet being low in digestible carbohydrate and thus leading to ketosis, but also to being low in indigestible fiber and thus starving certain gut bacteria.
Or, to phrase the same hypothesis differently, maybe one mechanism by which a glycolytic or high-fiber diet causes health problems is that it feeds harmful gut bacteria, and the presence of those bacteria causes the health problems.
If that hypothesis were true, it would imply that if you are eating a low-carb diet, then including a lot of low-carb vegetables would feed these hypothesized harmful gut bacteria and reduce some of the potential health benefits of a low-carb diet.
in brief
The purpose of this article is two-fold:
- First, to compare the authors’ interpretations of the observations to ours, given what we know about the metabolic effects of ketogenic diets.We draw attention to the fact that the metabolism of germ-free mice is strikingly similar to that of ketogenic dieters. This similarity holds at the whole-body level in terms of behaviour and physical characteristics, as well as the level of mitochondrial energetics. We show that these characteristics appear to be beneficial.
- Second, to raise the following questions:Are some of the benefits of a ketogenic diet mediated by starving gut bacteria, and if so, does eating fiber (i.e. low-carb vegetables) reduce some of the health benefits of a keto diet? Would eating a carbohydrate- and fiber- free diet confer some keto-like benefits even in the absence of ketosis?
the end of the chain
It all started when we saw the following statement on the Wikipedia page about butyrate :
“Butyrates are important as food for cells lining the mammalian colon (colonocytes). Without butyrates for energy, colon cells undergo autophagy (self digestion) and die.[1] Short-chain fatty acids, which include butyrate, are produced by beneficial colonic bacteria (probiotics) that feed on, or ferment prebiotics, which are plant products that contain adequate amounts of dietary fiber.”
The topic of butyrate is exciting to some scientists, because they have the idea that eating indigestible fiber is good for human health. Epidemiological studies have found correlations between high fiber intake and relatively less disease. However, randomised controlled trials have repeatedly failed to confirm the hypotheses that the fiber intake was actually protective. [1]. Therefore when a new idea comes up that might explain how eating fiber would be good for human health, scientists still hoping for such evidence latch onto it. Butyrate is an example of such a candidate mechanism for how eating fiber would be good for human health.
To us, since we think that eating fiber is useless (at best) for health, the statement above poses a challenge and a mystery. Almost the only source of butyrate in the human body is, as the wikipedia page explains in the excerpt above, from gut bacteria digesting fiber that you ate. (There’s also some butyrate in butter, presumably made the same way in cows.)
If butyrate is necessary for the health and even the survival of colon cells, wouldn’t that mean that a low-fiber diet — such as an all-meat diet — would be very unhealthy? Amber hasn’t eaten any significant amount of indigestible fiber in more than four years; does this mean that her colon cells have died off?
So we set out to investigate what led to that statement on wikipedia. Our investigation ultimately led to an intriguing hypothesis about a candidate mechanism to explain some of the health benefits of a keto diet.
The paper referenced as “[1]” on the wikipedia page is Donohoe-2011-“The Microbiome and Butyrate Regulate Energy Metabolism and Autophagy in the Mammalian Colon”. We read it with interest.
The authors of this paper performed a good experiment, made precise measurements, got interesting results, and clearly reported their results. But when it came to interpretation, they started with some assumptions we don’t think are warranted, and therefore produced a chain of reasoning that eventually led them and their readers, such as the authors of the wikipedia page, to conclusions that are opposite from ours.
other links in the chain
The paper describes an experiment in which they compare the metabolic mechanisms of “germ free” mice — mice that have been raised from birth without ever being allowed to contact common gut bacteria — with “conventional” mice. “Conventional” mice are genetically identical to the germ-free mice, but the researchers allow the conventional mice to be colonized by the bacteria that colonize all lab mice that aren’t specially isolated.
The authors cite previous experimenters who have raised germ-free mice and compared them to conventional mice. Those previous experiments compared body weight of the mice, how active they were, how much food they ate, and levels of different hormones and substances in their blood.
Here is their summary:
“[Germ-free] mice must consume 10–30% more food to maintain the same body weight as conventional controls. Despite this increased food intake, germ-free mice are leaner with a ~40% decrease in the size of their epididymal fat pads. They have a similar decrease in liver glycogen levels. [Germ-free] mice also have lower blood glucose and insulin levels and are resistant to obesity induced by a high-fat diet.” — from Donohoe-2011
The authors view this as evidence of detrimental metabolic inefficiency. They also note differences in metabolic workings of the mitochondria in the colon cells of the mice that they believe account for the inefficiency. Therefore they construct an experiment to discover whether feeding butyrate to the colon cells of the germ-free mice would restore them to working the way conventional colon cells work (which it does).
To us, on the other hand, the germ-free mouse sounds like the healthier mouse!
similarities between germ-free mice and ketogenic dieters
If you have followed the debates about low-carb diets conferring a metabolic advantage (demonstrated by their superior performance as weight loss diets [2], [3]) then the above description of germ-free mice should sound familiar. Compared to low-fat dieters, ketogenic dieters tend to be leaner (i.e. have a higher ratio of muscle to body fat), and have lower insulin and blood glucose levels. This can happen despite similar caloric intake. Their liver glycogen levels are also lower; ketogenesis may depend on low glycogen levels [4].
However, the germ-free mice are not ketogenic, presumably because they are consuming regular, glucose-plentiful diets. In fact they are less ketogenic than the conventional mice, as measured by beta-hydroxybutyrate in the blood.
So what is the cause of the similarity in metabolism between germ-free mice and ketogenic humans?
There is one other important way in which the germ-free mice were different from the conventional mice. They had lower NADH/NAD+ ratios and ATP levels (per mitochondrion) in their colon cells, but not in the liver, heart, or kidneys. Note that the heart, liver and kidneys favour fat metabolism, even in glycolytic (non-ketogenic) dieters [5].
The authors took this to be further evidence that the mice were in a state of energy deprivation, even though by all accounts they appeared to be using substantially more energy. They even previously mentioned this in connection with the reduced fatness:
“[Germ-free] mice exhibit increased locomotor activity. Therefore, the increased food consumption and decreased body fat of germ-free mice may simply be due to increased energy expenditure.” — from Donohoe-2011
However, it is a mistake to assume that lower NADH/NAD+ ratios and ATP levels per mitochondrion corresponds to less cellular energy. In fact, it is likely to be the opposite.
mitochondrial energetics is the commonality
There are three other conditions we know of that reduce the NADH/NAD+ ratio: calorie restriction [6], ketogenic diets [7], and the diabetes drug metformin [8]. Ketogenic diets share mechanisms with caloric restriction. Indeed, it seems likely that benefits of calorie restriction come from the activation of ketone bodies [9].
When you use fat and ketones for fuel instead of glucose, you produce fewer free radicals through reducing the NADH/NAD+ ratio [6]. This is probably the main mechanism by which it achieves the neuroprotection we mentioned in a recent post. Similarly, the reduction of the NADH/NAD+ ratio is probably one of the mechanisms by which calorie restriction can increase lifespan [5].
Calorie restriction also preserves ATP production, but it does this by increasing the number of mitochondria to match or exceed the lower ATP yield per mitochondrion [10], [11]. There is preliminary evidence that a ketogenic diet also increases mitochondrial number [12], [13]. So the idea of Donohoe et al. — that total ATP production is compromised because the NADH/NAD+ ratio in the individual mitochondrion has lowered ATP output per mitochondrion — seems unwarranted. Instead, there is likely to be a compensatory increase in mitochondrial number. That would be consistent with the fact that the mice appear to have more energy, not less. Ketogenic dieters have also been measured to have more energy expenditure than low-fat dieters [14].
So, a reduction of NADH/NAD+ ratio is associated with health benefits, and proposed longevity mechanisms. As you might now suspect, previous studies have shown that germ-free animals have increased lifespans [15]. (They also show decreased anxiety [16] and increased bone mass [17]. Once again, to us this sounds like a better kind of mouse to be!)
fiber-free for better health?
Given this observation — that some of the benefits of ketogenic diets are present in mice that don’t have gut bacteria which process dietary fiber, even though the mice are not in ketosis — it raises the following questions:
- Could the starvation of gut bacteria be a part of the mechanism of the benefits of ketogenic diets?
- Since butyrate restores the mitochondrial working of the cells to be like the conventional controls (which, from our perspective, is a worse physiological state), could fiber be actually counter-productive to a ketogenic diet?
- In analogy to the way the putative benefits of fiber may simply be that they displace refined carbohydrates in the diet, could the reason probiotics can lead to improved health be not because they are beneficial, but because they push out more harmful strains [18]?
- Could a diet free of carb and fiber (i.e. one extremely low in plants) have benefits independent of its tendency to be ketogenic?
We don’t have enough evidence to settle these questions, but they are interesting hypotheses that come directly from the results of this study.
in sum
- Contrary to the conclusions of the authors and Wikipedia editors that butyrate is necessary for cell energy, we interpret the results as showing improved cellular energy in the absence of butyrate.
- We now have another source for making hypotheses about potentially important cellular metabolism. Before we learned about germ free mice, we could already use mechanisms discovered from caloric restriction and compare them to mechanisms of ketogenic diets. Now we can compare and contrast mechanisms from caloric restriction, ketogenic diets, and germ-free animals.
- This raises some interesting (perhaps even provocative) questions about the health effects of dietary fiber and gut flora on human health.
notes
1. Evidence type: review
The hot air and cold facts of dietary fibre.
Carla S Coffin, MD FRCPC and Eldon A Shaffer, MD FRCPC
Can J Gastroenterol. 2006 April; 20(4): 255–256.
(emphasis ours)
“A recent pooled analysis of 13 prospective cohort studies (6) found that dietary fibre was not associated with a reduced risk of colorectal cancer after adjusting for other dietary risk factors. The Cochrane collaboration (7) systematically reviewed five studies of over 4000 subjects for the effect of dietary fibre on the incidence or recurrence of colorectal adenomas and incidence of colorectal cancer over a two-to four-year period. The population included all subjects that had adenomatous polyps but no history of colorectal cancer or a documented ‘clean colon’ at baseline with follow-up colonoscopy. Study interventions included soluble and insoluble dietary fibre or a comprehensive dietary intervention with high fibre whole food sources. The combined data showed no outcome difference between the intervention and control groups in the number of subjects with at least one adenoma or a new diagnosis of colorectal cancer. The Cochrane reviewers (7) concluded that there was no evidence from randomized controlled trials to suggest that increased dietary fibre intake would reduce the incidence or recurrence of adenomatous polyps.
“Widespread popular media advertisements have purported the benefits of soluble fibre in lowering the risk of atherosclerotic coronary artery disease, mainly by modifying the main coronary artery disease risk factors (ie, dyslipidemia, diabetes and obesity). As for diabetes, high fibre diets slow the postprandial rise in blood glucose and thus, improve glycemic control (8). In dyslipidemic patients, pundits have proposed that psyllium lowers serum cholesterol by binding bile acids in the intestinal lumen resulting in decreased absorption and increased fecal excretion. The ensuing bile acid depletion increases hepatic demand for the de novo synthesis of bile acids from cholesterol. Investigating this mechanism, Van Rosendaal et al (9) found that fibre administration had no effect and certainly did not lower serum cholesterol. Similarly, an earlier study (10) comparing the effect of wheat bran on serum cholesterol of hyperlipidemic and normolipidemic controls showed no change in total cholesterol or ratio of low density lipoprotein to high density lipoprotein cholesterol. Another trial (11) of intensive dietary advice regarding fat, cereal fibre and fish intake on diet and mortality of men with a recent history of myocardial infarction did not find any substantial long-term benefit. The authors admitted to limitations of dietary data in the study (ie, only short-term period of advice and limited number of questions), but there was no evidence to guide decisions about value of dietary advice to increase fish or cereal fibre by people with coronary disease. We await the results of three Cochrane protocols undertaken to review the evidence of dietary fibre in fruits and vegetables, wholegrain cereals or high-fat, low fibre dietary intervention in the prevention of coronary heart disease (12–14). Any conclusions regarding the effectiveness of fibre for the prevention of heart disease appear premature.”
[…]
“In one of the first randomized, placebo-controlled trials of the role of bran in patients with [diverticular disease] (17), the authors concluded that dietary fibre supplements do nothing more than relieve constipation, and the impression that fibre helps [diverticular disease] is “simply a manifestation of western civilization’s obsession with the need for frequent defecation”. Recent systematic reviews (18,19) of the role of dietary fibre and [diverticular disease] (both asymptomatic diverticulosis and symptomatic diverticulitis) conclude that most of the positive evidence of the effects of fibre supplementation in treating or preventing disease is from retrospective analyses with inherent limitations and high risk of bias.”
[…]
“Systematic reviews have shown that the treatment of IBS patients with fibre is controversial. One recent meta-analysis of 17 randomized controlled trials (20) quantified the effectiveness of different types of fibre. The reviewers found that fibre was only marginally effective in terms of global symptom improvement or constipation and there was no effect in IBS related abdominal pain. Fibre has a role in treating constipation but its value for IBS, pain and diarrhea is controversial. Any effectivenss of fibre in the long-term management of IBS remains questionable. Clinically, bran is no better than placebo in the relief of the overall symptoms of IBS, and is possibly worse than a normal diet for some symptoms.”
2. Evidence type: review of randomised controlled trials in humans.
Systematic review of randomized controlled trials of low-carbohydrate vs. low-fat/low-calorie diets in the management of obesity and its comorbidities.
Hession M, Rolland C, Kulkarni U, Wise A, Broom J.
Obes Rev. 2009 Jan;10(1):36-50. doi: 10.1111/j.1467-789X.2008.00518.x. Epub 2008 Aug 11.
“Abstract
There are few studies comparing the effects of low-carbohydrate/high-protein diets with low-fat/high-carbohydrate diets for obesity and cardiovascular disease risk. This systematic review focuses on randomized controlled trials of low-carbohydrate diets compared with low-fat/low-calorie diets. Studies conducted in adult populations with mean or median body mass index of > or =28 kg m(-2) were included. Thirteen electronic databases were searched and randomized controlled trials from January 2000 to March 2007 were evaluated. Trials were included if they lasted at least 6 months and assessed the weight-loss effects of low-carbohydrate diets against low-fat/low-calorie diets. For each study, data were abstracted and checked by two researchers prior to electronic data entry. The computer program Review Manager 4.2.2 was used for the data analysis. Thirteen articles met the inclusion criteria. There were significant differences between the groups for weight, high-density lipoprotein cholesterol, triacylglycerols and systolic blood pressure, favouring the low-carbohydrate diet. There was a higher attrition rate in the low-fat compared with the low-carbohydrate groups suggesting a patient preference for a low-carbohydrate/high-protein approach as opposed to the Public Health preference of a low-fat/high-carbohydrate diet. Evidence from this systematic review demonstrates that low-carbohydrate/high-protein diets are more effective at 6 months and are as effective, if not more, as low-fat diets in reducing weight and cardiovascular disease risk up to 1 year. More evidence and longer-term studies are needed to assess the long-term cardiovascular benefits from the weight loss achieved using these diets.”
3.3. Evidence type: review of randomised controlled trials in humans.
Although this analysis is not peer-reveiwed, it is thorough, appears to be accurate, and does not omit any counter-evidence as far as we are aware.
23 Studies on Low-Carb and Low-Fat Diets – Time to Retire The Fad
Kris Gunnars
October 15, 2013
Authority Nutrition
(emphasis ours)
“In this article, I have analyzed the data from 23 of these studies comparing low-carb and low-fat diets.
“All of the studies are randomized controlled trials, the gold standard of science. All are published in respected, peer-reviewed journals.
…
“The majority of studies achieved statistically significant differences in weight loss (always in favor of low-carb). There are several other factors that are worth noting:
- The low-carb groups often lost 2-3 times as much weight as the low-fat groups. In a few instances there was no significant difference.
- In most cases, calories were restricted in the low-fat groups, while the low-carb groups could eat as much as they wanted.
- When both groups restricted calories, the low-carb dieters still lost more weight (7, 13, 19), although it was not always significant (8, 18, 20).
- There was only one study where the low-fat group lost more weight (23) although the difference was small (0.5 kg – 1.1 lb) and not statistically significant.
- In several of the studies, weight loss was greatest in the beginning. Then people start regaining the weight over time as they abandon the diet.
- When the researchers looked at abdominal fat (the unhealthy visceral fat) directly, low-carb diets had a clear advantage (5, 7, 19).
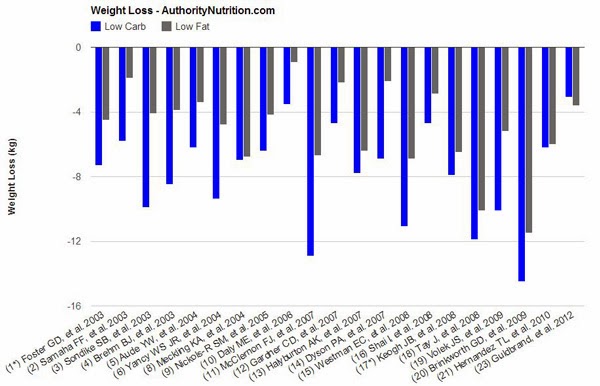
4. Evidence type: review of experiments
Hormonal control of ketogenesis. Biochemical considerations.
McGarry JD, Foster DW.
Arch Intern Med. 1977 Apr;137(4):495-501.
“Abstract
A two-site, bihormonal concept for the control of ketone body production is proposed. Thus, ketosis is viewed as the result of increased mobilization of free fatty acids from adipose tissue (site 1) to the liver (site 2), coupled with simultaneous enhancement of the liver’s capacity to convert these substrates into acetoacetic and beta-hydroxybutyric acids. The former event is believed to be triggered by a fall in plasma insulin levels while the latter is considered to be effected primarily by the concomitant glucagon excess characteristic of the ketotic state. Although the precise mechanism whereby elevation of the circulating [glucagon]:[insulin] ratio stimulates hepatic ketogenic potential is not known, activation of the carnitine acyltransferase reaction, the first step in the oxidation of fatty acids, is an essential feature. Two prerequisites for this metabolic adaptation in liver appear to be an elevation in its carnitine content and depletion of its glycogen stores. Despite present limitations the model (evolved mainly from rat studies) provides a framework for the description of various types of clinical ketosis in biochemical terms and may be useful for future studies.”
5. Evidence type: authority
Dynamic Adaptation of Nutrient Utilization in Humans.
El Bacha, T., Luz, M. & Da Poian, A. (2010)
Nature Education 3(9):8
“[M]any different cells do oxidize fatty acids for ATP production. Between meals, cardiac muscle cells meet 90% of their ATP demands by oxidizing fatty acids. Although these proportions may fall to about 60% depending on the nutritional status and the intensity of contractions, fatty acids may be considered the major fuel consumed by cardiac muscle.
…
Other organs that use primarily fatty acid oxidation are the kidney and the liver.”
6. Evidence type: controlled non-human animal experiments
Calorie restriction extends yeast life span by lowering the level of NADH.
Su-Ju Lin, Ethan Ford, Marcia Haigis, Greg Liszt, and Leonard Guarente.
Genes Dev. 2004 January 1; 18(1): 12–16.
“Our studies show that a switch to oxidative metabolism during CR increases the NAD/NADH ratio by decreasing NADH levels. NADH is a competitive inhibitor of Sir2, implying that a reduction in this dinucleotide activates Sir2 to extend the life span in CR. Indeed, overexpression of the NADH dehydrogenase specifically lowers NADH levels and extends the life span, providing strong support for this hypothesis. Regulation of the life span by NADH is also consistent with the earlier finding that electron transport is required for longevity during CR (Lin et al. 2002). The NAD/NADH ratio reflects the intracellular redox state and is a readout of metabolic activity. Our findings suggest that this ratio can serve a critical regulatory function, namely, the determination of the life span of yeast mother cells. It remains to be seen whether this ratio will serve related regulatory functions in higher organisms.”
7. Evidence type: controlled non-human animal experiments
Ketones inhibit mitochondrial production of reactive oxygen species production following glutamate excitotoxicity by increasing NADH oxidation.
Marwan Maalouf, Patrick G. Sullivan, Laurie Davis, Do Young Kim, and Jong M. Rho Neuroscience.
2007 March 2; 145(1): 256–264.
“[W]e demonstrate that ketones reduce glutamate-induced free radical formation by increasing the NAD+/NADH ratio and enhancing mitochondrial respiration in neocortical neurons. This mechanism may, in part, contribute to the neuroprotective activity of ketones by restoring normal bioenergetic function in the face of oxidative stress.”
8. Evidence type: controlled non-human animal experiment
Metformin suppresses hepatic gluconeogenesis through induction of SIRT1 and GCN5
Paul W Caton, Nanda K Nayuni, Julius Kieswich, Noorafza Q Khan, Muhammed M Yaqoob and Roger Corder
J Endocrinol April 1, 2010 205 97-106
(emphasis ours)
“Results
Metformin increases SIRT1 in db/db mice
Systemic activation of SIRT1 with the activator SRT1720 is reported to lower blood glucose and improve insulin sensitivity in Zucker rats and diet-induced obese mice in part through inhibition of hepatic gluconeogenesis (Milne et al. 2007). Therefore, we investigated whether metformin inhibited gluconeogenesis through changes in hepatic SIRT1. Eight-week-old db/db or control (db/m) mice were administered metformin (250 mg/kg per day; 7 days). Levels of SIRT1 protein, activity and NAD+/NADH ratio were significantly increased in metformin-treated db/db mice compared with the controls and untreated db/db mice (Fig. 1A, C and D). Despite increased protein levels, Sirt1 mRNA levels were unchanged following metformin treatment (Fig. 1B). Levels of SIRT1 protein and activity as well as NAD+/NADH levels were unchanged between the control and untreated mice (Fig. 1A–C). Metformin had no effect on SIRT1 in control mice (data not shown). Furthermore, incubation of HepG2 cells with metformin (2 mM) also resulted in increased levels of SIRT1 protein and activity and NAD+/NADH ratio (Fig. 1E–G). This indicates that increasing SIRT1 protein and activity could be a key mechanism by which metformin inhibits gluconeogenic gene expression.”
9. Evidence type: review of experiments
The neuroprotective properties of calorie restriction, the ketogenic diet, and ketone bodies.
Marwan A. Maalouf, Jong M. Rho, and Mark P. Mattson
Brain Res Rev. 2009 March; 59(2): 293–315.
“Calorie restriction and the ketogenic diet share two characteristics: reduced carbohydrate intake and a compensatory rise in ketone bodies. The neuroprotective effects of reduced carbohydrate per se are being investigated by several research groups (Mattson et al. 2003; Ingram et al. 2006). We have evaluated the possibility that ketone bodies might mediate the neuroprotective effects of calorie restriction and of the ketogenic diet. An expanding body of evidence indicates that ketone bodies are indeed neuroprotective and that the underlying mechanisms are similar to those associated with calorie restriction – specifically at the mitochondrial level.”
10. Evidence type: review of clinical reports
Calorie restriction induces mitochondrial biogenesis and bioenergetic efficiency.
G. López-Lluch, N. Hunt, B. Jones, M. Zhu, H. Jamieson, S. Hilmer, M. V. Cascajo, J. Allard, D. K. Ingram, P. Navas, and R. de Cabo
Proc Natl Acad Sci U S A. 2006 February 7; 103(6): 1768–1773.
“[M]itochondria under CR conditions show less oxygen consumption, reduce membrane potential, and generate less reactive oxygen species than controls, but remarkably they are able to maintain their critical ATP production. In effect, CR can induce a peroxisome proliferation-activated receptor coactivator 1α-dependent increase in mitochondria capable of efficient and balanced bioenergetics to reduce oxidative stress and attenuate age-dependent endogenous oxidative damage.”
11. Evidence type: review of controlled experiments
The neuroprotective properties of calorie restriction, the ketogenic diet, and ketone bodies
Marwan A. Maalouf, Jong M. Rho, and Mark P. Mattson
Brain Res Rev. 2009 March; 59(2): 293–315.
(emphasis ours)
“Slowing of brain aging in calorie-restricted animals was originally believed to result from reduced metabolic activity and, hence, decreased production of reactive oxygen species, a natural byproduct of oxidative metabolism (Wolf 2006). Several studies revealed that calorie restriction was associated with energy conservation (Gonzales-Pacheco et al. 1993; Santos-Pintos et al. 2001) and that mitochondria isolated from calorie-restricted animals produced less ATP than those from controls fed ad libitum, a finding compatible with increased UCP activity (Sreekumar et al. 2002; Drew et al. 2003). However, separate investigations in rodents have suggested that, when adjusted for body weight, metabolic rate does not decrease with calorie restriction (Masoro et al. 1982; McCarter et al. 1985; Masoro 1993). More importantly, calorie restriction prevents the age-related decline in oxidative metabolism in muscle (Hepple et al. 2005; Baker et al. 2006). These data are supported by recent studies indicating that, in contrast to isolated mitochondria, ATP synthesis in intact myocytes and in vivo does not decrease following calorie restriction (Lopez-Lluch et al. 2006; Zangarelli et al. 2006). Additional support is provided by the finding that, in yeast, oxidative metabolism increases with calorie restriction (Lin et al. 2002). […] Although the effects of calorie restriction on ATP generation might appear to contradict those on uncoupling proteins, this discrepancy can be explained by the fact that calorie restriction also promotes mitochondrial biogenesis, thereby enhancing total metabolic output per cell while decreasing mitochondrial production of reactive oxygen species (Diano et al. 2003; Nisoli et al. 2005; Civitarese et al. 2007).”
12. Evidence type: in vitro non-human animal experiment
Mitochondrial biogenesis in the anticonvulsant mechanism of the ketogenic diet.
Bough KJ, Wetherington J, Hassel B, Pare JF, Gawryluk JW, Greene JG, Shaw R, Smith Y, Geiger JD, Dingledine RJ.
Ann Neurol. 2006 Aug;60(2):223-35.
“OBJECTIVE
The full anticonvulsant effect of the ketogenic diet (KD) can require weeks to develop in rats, suggesting that altered gene expression is involved. The KD typically is used in pediatric epilepsies, but is effective also in adolescents and adults. Our goal was to use microarray and complementary technologies in adolescent rats to understand its anticonvulsant effect.
METHODS
Microarrays were used to define patterns of gene expression in the hippocampus of rats fed a KD or control diet for 3 weeks. Hippocampi from control- and KD-fed rats were also compared for the number of mitochondrial profiles in electron micrographs, the levels of selected energy metabolites and enzyme activities, and the effect of low glucose on synaptic transmission.
RESULTS
Most striking was a coordinated upregulation of all (n = 34) differentially regulated transcripts encoding energy metabolism enzymes and 39 of 42 transcripts encoding mitochondrial proteins, which was accompanied by an increased number of mitochondrial profiles, a higher phosphocreatine/creatine ratio, elevated glutamate levels, and decreased glycogen levels. Consistent with increased energy reserves, synaptic transmission in hippocampal slices from KD-fed animals was resistant to low glucose.
CONCLUSION
These data show that a calorie-restricted KD enhances brain metabolism. We propose an anticonvulsant mechanism of the KD involving mitochondrial biogenesis leading to enhanced alternative energy stores.”
13.Evidence type: controlled non-human animal experiments
Mitochondrial biogenesis and increased uncoupling protein 1 in brown adipose tissue of mice fed a ketone ester diet.
Srivastava S, Kashiwaya Y, King MT, Baxa U, Tam J, Niu G, Chen X, Clarke K, Veech RL.
FASEB J. 2012 June; 26(6): 2351–2362.
(Emphasis ours)
Abstract
“We measured the effects of a diet in which d-β-hydroxybutyrate-(R)-1,3 butanediol monoester [ketone ester (KE)] replaced equicaloric amounts of carbohydrate on 8-wk-old male C57BL/6J mice. Diets contained equal amounts of fat, protein, and micronutrients. The KE group was fed ad libitum, whereas the control (Ctrl) mice were pair-fed to the KE group. Blood d-β-hydroxybutyrate levels in the KE group were 3-5 times those reported with high-fat ketogenic diets. Voluntary food intake was reduced dose dependently with the KE diet. Feeding the KE diet for up to 1 mo increased the number of mitochondria and doubled the electron transport chain proteins, uncoupling protein 1, and mitochondrial biogenesis-regulating proteins in the interscapular brown adipose tissue (IBAT). [18F]-Fluorodeoxyglucose uptake in IBAT of the KE group was twice that in IBAT of the Ctrl group. Plasma leptin levels of the KE group were more than 2-fold those of the Ctrl group and were associated with increased sympathetic nervous system activity to IBAT. The KE group exhibited 14% greater resting energy expenditure, but the total energy expenditure measured over a 24-h period or body weights was not different. The quantitative insulin-sensitivity check index was 73% higher in the KE group. These results identify KE as a potential antiobesity supplement.”
14. Evidence type: randomised controlled clinical trial
Effects of Dietary Composition on Energy Expenditure During Weight-Loss Maintenance
Cara B. Ebbeling, PhD; Janis F. Swain, MS, RD; Henry A. Feldman, PhD; William W. Wong, PhD; David L. Hachey, PhD; Erica Garcia-Lago, BA; David S. Ludwig, MD, PhD
JAMA. 2012;307(24):2627-2634. doi:10.1001/jama.2012.6607.
“The results of our study challenge the notion that a calorie is a calorie from a metabolic perspective. During isocaloric feeding following weight loss, REE was 67 kcal/d higher with the very low-carbohydrate diet compared with the low-fat diet. TEE differed by approximately 300 kcal/d between these 2 diets, an effect corresponding with the amount of energy typically expended in 1 hour of moderate-intensity physical activity.”
15. Evidence type: review of non-human animal experiments
The gnotobiotic animal as a tool in the study of host microbial relationships.
H A Gordon and L Pesti.
Bacteriol Rev. 1971 December; 35(4): 390–429.
“Two attempts have been made to construct life tables and to determine lesions at natural death in germ-free and conventional animals. One study (105) was conducted in genetically closely linked Swiss Webster mice and included over 300 germ-free and the same number of conventional controls which were introduced into the colony at the age of 12 months (to eliminate the effect of early losses). At natural death, the ages of the mice were (means and standard errors in days 19; females, are given): germ-free males, 723 681 i 12; conventional males, 480 i 10; females, 516 i 10. This pattern of survival rates seemed to continue throughout the course of the experi- ment. In the second study (335), approximately 50 germ-free and the same number of conven- tional ICR mice were introduced into the colony after weaning. At natural death, the age of the mice was (using the same mode of expression): germ-free males, 556 i 43; females, 535 + 46; 41; females, 547 ± conventional males, 536 45. In the first trimester of life, the survival rate was essentially the same in the germ-free and conventional control groups. In the middle third, the germ-free mice displayed an increased survival rate (e.g., 40% cumulative mortality was reached for the combined group of germ-free males and females only at the age of approximately 580 days, whereas for the conventional controls this value was approximately 410 days). Increased mortality of the germ-free group at more advanced age resulted in the similarity of mean ages between the opposing animal groups when all animals participating in the study were considered.”
16. Evidence type: controlled non-human animal experiment
Normal gut microbiota modulates brain development and behavior.
Rochellys Diaz Heijtz, Shugui Wang, Farhana Anuar, Yu Qian, Britta Björkholm, Annika Samuelsson, Martin L. Hibberd, Hans Forssberg, and Sven Pettersson
Proc Natl Acad Sci U S A. 2011 February 15; 108(7): 3047–3052.
“Microbial colonization of mammals is an evolution-driven process that modulate host physiology, many of which are associated with immunity and nutrient intake. Here, we report that colonization by gut microbiota impacts mammalian brain development and subsequent adult behavior. Using measures of motor activity and anxiety-like behavior, we demonstrate that germ free (GF) mice display increased motor activity and reduced anxiety, compared with specific pathogen free (SPF) mice with a normal gut microbiota. This behavioral phenotype is associated with altered expression of genes known to be involved in second messenger pathways and synaptic long-term potentiation in brain regions implicated in motor control and anxiety-like behavior. GF mice exposed to gut microbiota early in life display similar characteristics as SPF mice, including reduced expression of PSD-95 and synaptophysin in the striatum. Hence, our results suggest that the microbial colonization process initiates signaling mechanisms that affect neuronal circuits involved in motor control and anxiety behavior.”
17. Evidence type: controlled non-human animal experiment
The gut microbiota regulates bone mass in mice.
Sjögren K, Engdahl C, Henning P, Lerner UH, Tremaroli V, Lagerquist MK, Bäckhed F, Ohlsson C.
J Bone Miner Res. 2012 Jun;27(6):1357-67. doi: 10.1002/jbmr.1588.
“Abstract
The gut microbiota modulates host metabolism and development of immune status. Here we show that the gut microbiota is also a major regulator of bone mass in mice. Germ-free (GF) mice exhibit increased bone mass associated with reduced number of osteoclasts per bone surface compared with conventionally raised (CONV-R) mice. Colonization of GF mice with a normal gut microbiota normalizes bone mass. Furthermore, GF mice have decreased frequency of CD4(+) T cells and CD11b(+) /GR 1 osteoclast precursor cells in bone marrow, which could be normalized by colonization. GF mice exhibited reduced expression of inflammatory cytokines in bone and bone marrow compared with CONV-R mice. In summary, the gut microbiota regulates bone mass in mice, and we provide evidence for a mechanism involving altered immune status in bone and thereby affected osteoclast-mediated bone resorption. Further studies are required to evaluate the gut microbiota as a novel therapeutic target for osteoporosis.”
18. Evidence type: review of experiments and hypotheses
Probiotics and their fermented food products are beneficial for health.
Parvez S, Malik KA, Ah Kang S, Kim HY.
J Appl Microbiol. 2006 Jun;100(6):1171-85.
(emphasis ours)
“Abstract
Probiotics are usually defined as microbial food supplements with beneficial effects on the consumers. Most probiotics fall into the group of organisms’ known as lactic acid-producing bacteria and are normally consumed in the form of yogurt, fermented milks or other fermented foods. Some of the beneficial effect of lactic acid bacteria consumption include: (i) improving intestinal tract health; (ii) enhancing the immune system, synthesizing and enhancing the bioavailability of nutrients; (iii) reducing symptoms of lactose intolerance, decreasing the prevalence of allergy in susceptible individuals; and (iv) reducing risk of certain cancers. The mechanisms by which probiotics exert their effects are largely unknown, but may involve modifying gut pH, antagonizing pathogens through production of antimicrobial compounds, competing for pathogen binding and receptor sites as well as for available nutrients and growth factors, stimulating immunomodulatory cells, and producing lactase. Selection criteria, efficacy, food and supplement sources and safety issues around probiotics are reviewed. Recent scientific investigation has supported the important role of probiotics as a part of a healthy diet for human as well as for animals and may be an avenue to provide a safe, cost effective, and ‘natural’ approach that adds a barrier against microbial infection. This paper presents a review of probiotics in health maintenance and disease prevention.”